IQuS Publications
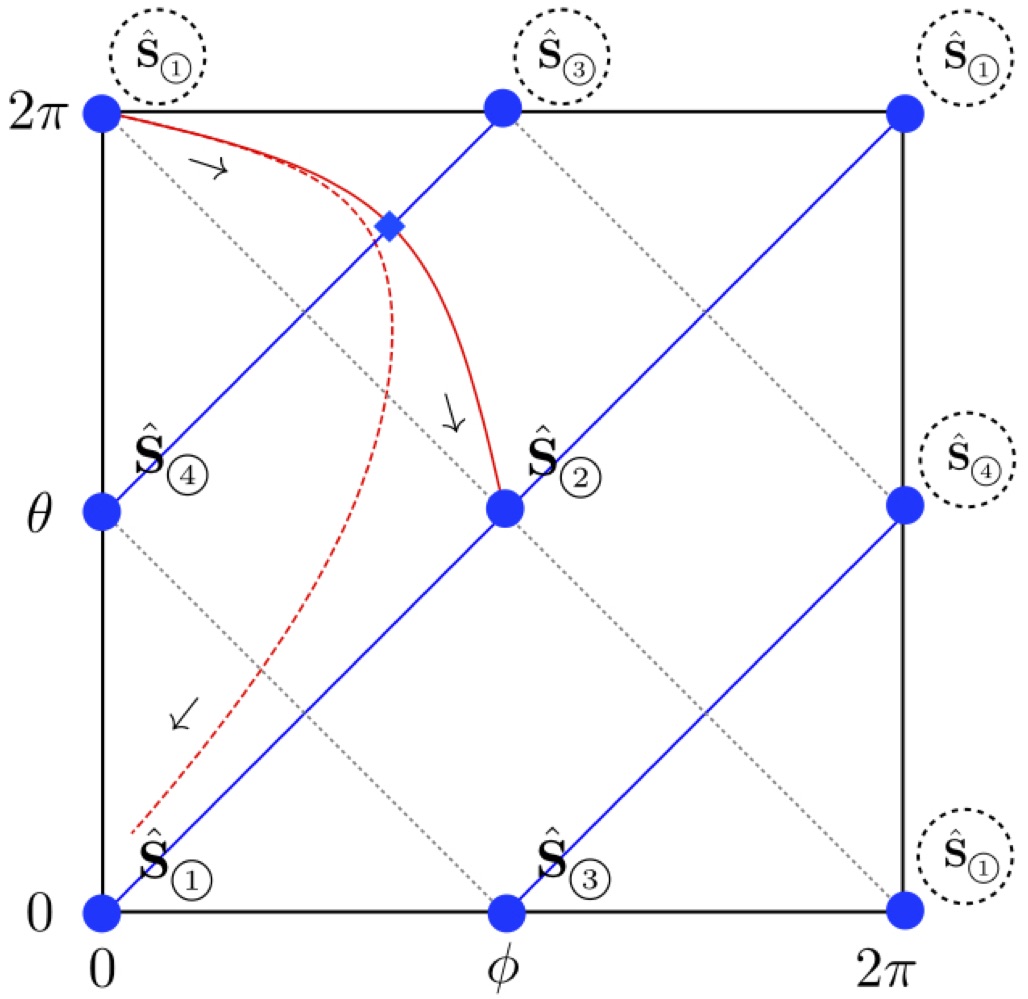
Geometry and entanglement in the scattering matrix
A formulation of nucleon-nucleon scattering is developed in which the S-matrix, rather than an effective-field theory (EFT) action, is the fundamental object. Spacetime plays no role in this description: the S-matrix is a trajectory that moves between RG fixed points in a compact theory space defined by unitarity. This theory space has a natural operator definition, and a geometric embedding of the unitarity constraints in four-dimensional Euclidean space yields a flat torus, which serves as the stage on which the S-matrix propagates. Trajectories with vanishing entanglement are special geodesics between RG fixed points on the flat torus, while entanglement is driven by an external potential. The system of equations describing S-matrix trajectories is in general complicated, however the very-low-energy S-matrix — that appears at leading-order in the EFT description — possesses a UV/IR conformal invariance which renders the system of equations integrable, and completely determines the potential. In this geometric viewpoint, inelasticity is in correspondence with the radius of a three-dimensional hyperbolic space whose two-dimensional boundary is the flat torus. This space has a singularity at vanishing radius, corresponding to maximal violation of unitarity. The trajectory on the flat torus boundary can be explicitly constructed from a bulk trajectory with a quantifiable error, providing a simple example of a holographic quantum error correcting code.
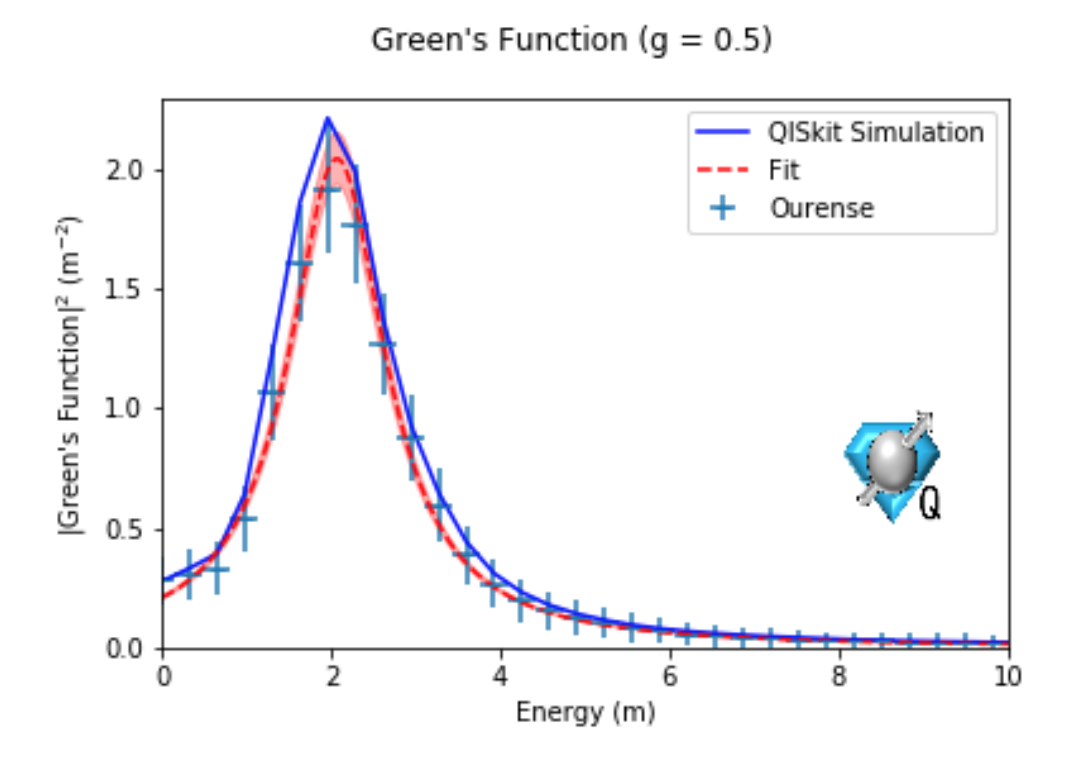
An Algorithm for Quantum Computation of Particle Decays
A quantum algorithm is developed to calculate decay rates and cross sections using quantum resources that scale polynomially in the system size assuming similar scaling for state preparation and time evolution. This is done by computing finite-volume one- and two-particle Green’s functions on the quantum hardware. Particle decay rates and two particle scattering cross sections are extracted from the imaginary parts of the Green’s function. A 0 + 1 dimensional implementation of this method is demonstrated on IBM’s superconducting quantum hardware for the decay of a heavy scalar particle to a pair of light scalars.
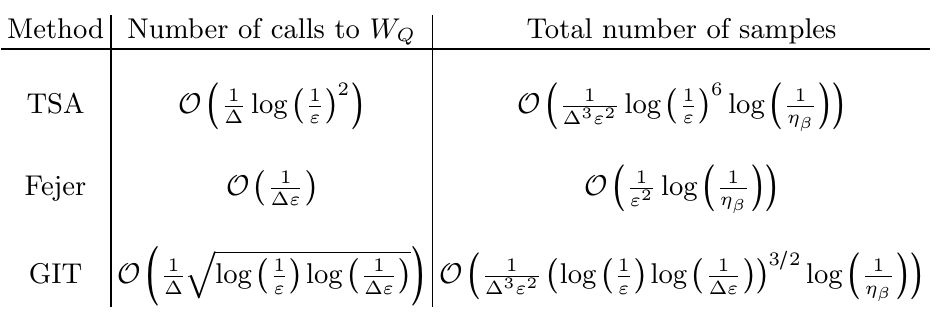
Spectral Density Estimation with the Gaussian Integral Transform
The spectral density operator ρ(ω)=δ(ω−H) plays a central role in linear response theory as it’s expectation value, the dynamical response function, can be used to compute scattering cross-sections. In this work, we describe a near optimal quantum algorithm providing an approximation to the spectral density with energy resolution Δ and error ϵ using O(√(log(1/ϵ)(log(1/Δ)+log(1/ϵ)))/Δ) operations. This is achieved without using expensive approximations to the time-evolution operator but exploiting instead qubitization to implement an approximate Gaussian Integral Transform (GIT) of the spectral density. We also describe appropriate error metrics to assess the quality of spectral function approximations more generally.
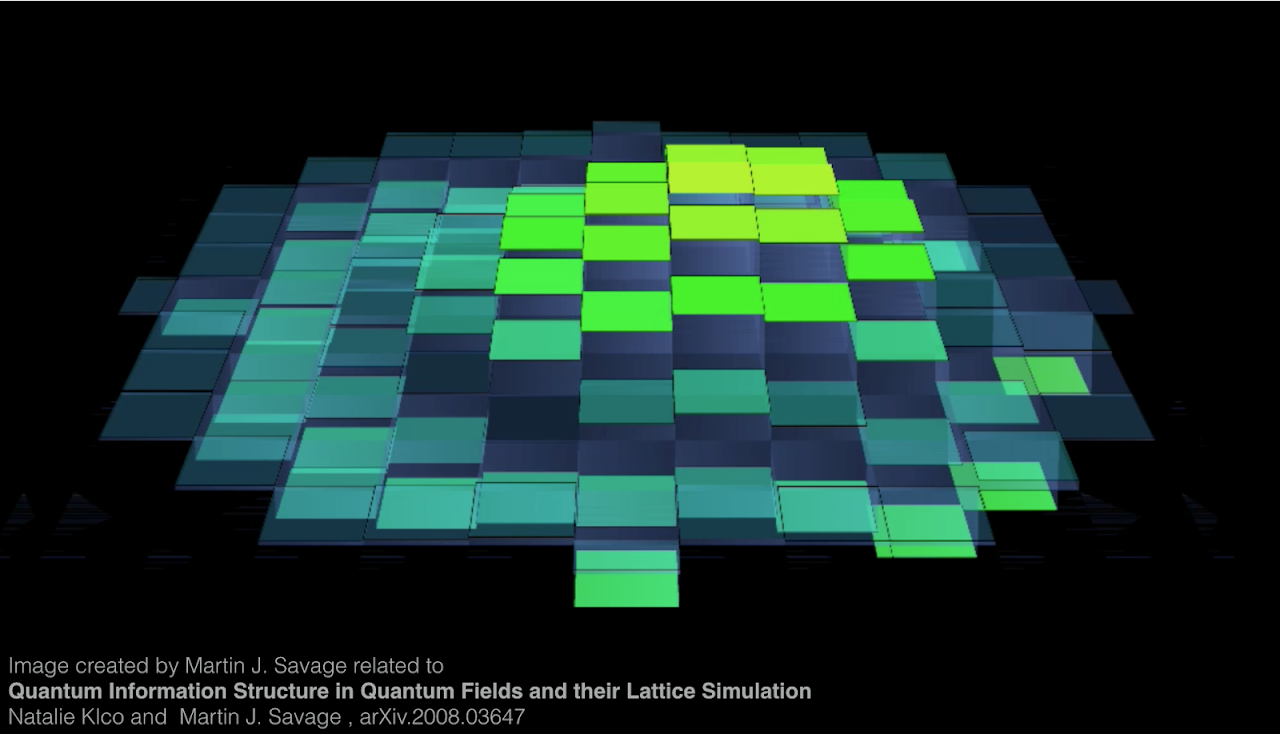
Geometric Quantum Information Structure in Quantum Fields and their Lattice Simulation
An upper limit to distillable entanglement between two disconnected regions of massless noninteracting scalar field theory has an exponential decay defined by a geometric decay constant. When regulated at short distances with a spatial lattice, this entanglement abruptly vanishes beyond a dimensionless separation, defining a negativity sphere. In two spatial dimensions, we determine this geometric decay constant between a pair of disks and the growth of the negativity sphere toward the continuum through a series of lattice calculations. Making the connection to quantum field theories in three-spatial dimensions, assuming such quantum information scales appear also in quantum chromodynamics (QCD), a new relative scale may be present in effective field theories describing the low-energy dynamics of nucleons and nuclei. We highlight potential impacts of the distillable entanglement structure on effective field theories, lattice QCD calculations and future quantum simulations.
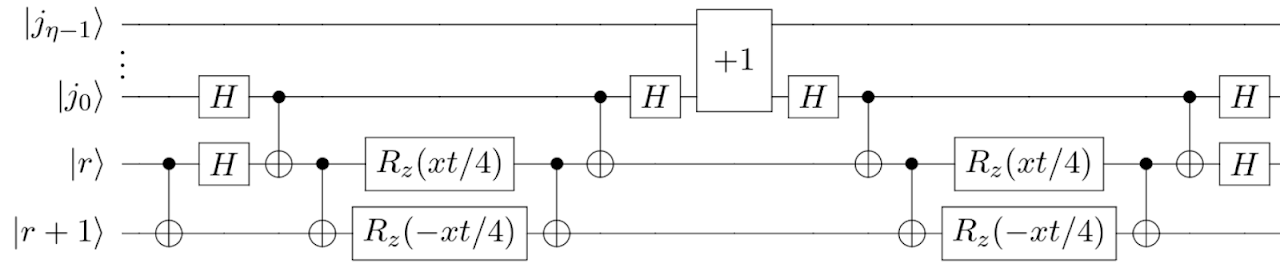
Quantum Algorithms for Simulating the Lattice Schwinger Model
The Schwinger model (quantum electrodynamics in 1+1 dimensions) is a testbed for the study of quantum gauge field theories. We give scalable, explicit digital quantum algorithms to simulate the lattice Schwinger model in both NISQ and fault-tolerant settings. In particular, we perform a tight analysis of low-order Trotter formula simulations of the Schwinger model, using recently derived commutator bounds, and give upper bounds on the resources needed for simulations in both scenarios. In lattice units, we find a Schwinger model on N/2 physical sites with coupling constant x-1/2 and electric field cutoff x-1/2Λ can be simulated on a quantum computer for time 2xT using a number of T-gates or CNOTs in Õ(N3/2 T3/2 x1/2 Λ) for fixed operator error. This scaling with the truncation Λ is better than that expected from algorithms such as qubitization or QDRIFT. Furthermore, we give scalable measurement schemes and algorithms to estimate observables which we cost in both the NISQ and fault-tolerant settings by assuming a simple target observable—the mean pair density. Finally, we bound the root-mean-square error in estimating this observable via simulation as a function of the diamond distance between the ideal and actual CNOT channels. This work provides a rigorous analysis of simulating the Schwinger model, while also providing benchmarks against which subsequent simulation algorithms can be tested.
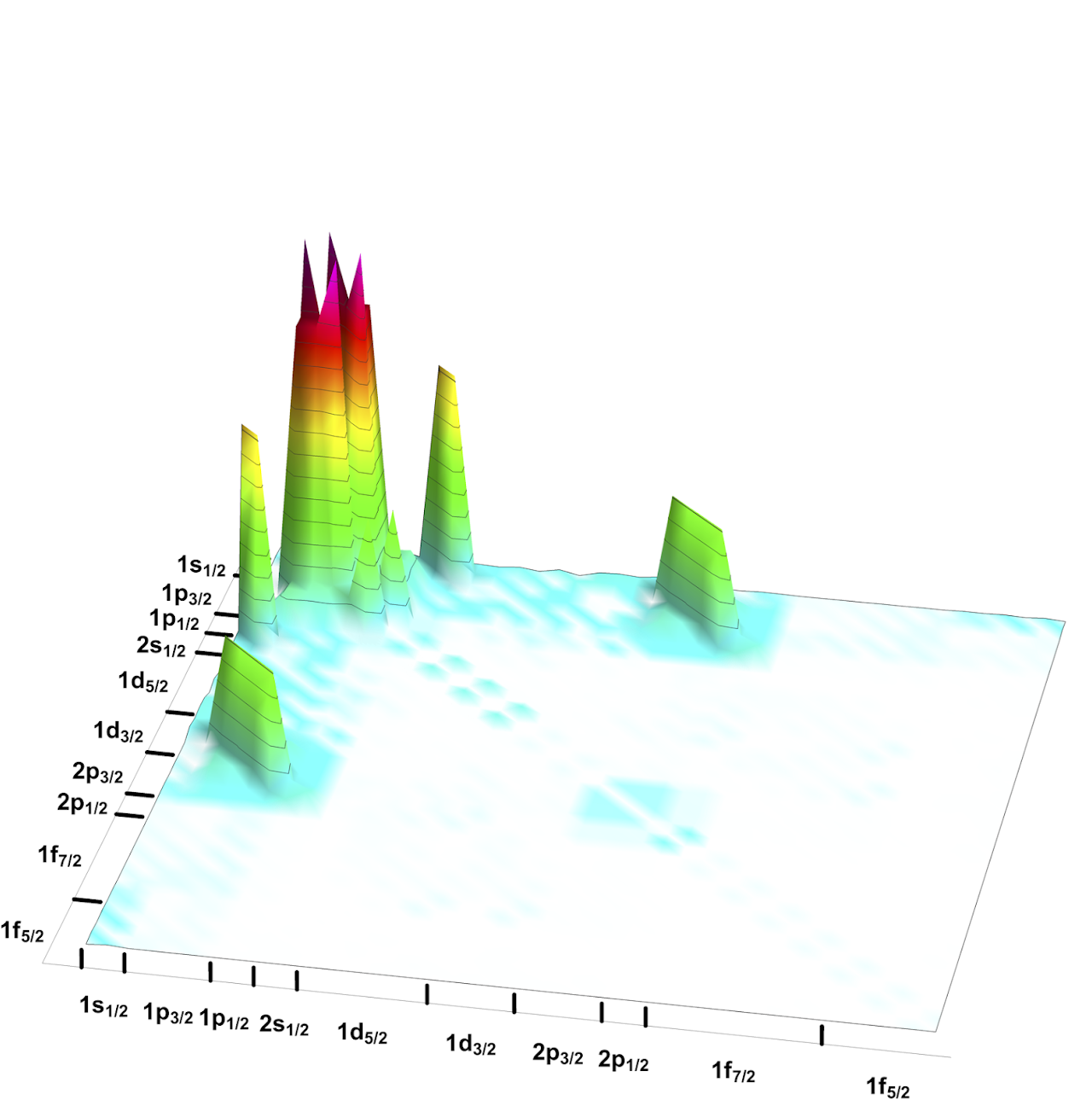
Entanglement Rearrangement in Self-Consistent Nuclear Structure Calculations
Entanglement properties of 4He and 6He are investigated using nuclear many-body calculations, specifically the single-nucleon entanglement entropy, and the two-nucleon mutual information and negativity. Nuclear wavefunctions are obtained by performing active-space no-core configuration interaction calculations using a two-body nucleon-nucleon interaction derived from chiral effective field theory. Entanglement measures within single-particle bases, the harmonic oscillator (HO), Hartree-Fock (HF), natural (NAT) and variational natural (VNAT) bases, are found to exhibit different degrees of complexity. Entanglement in both nuclei is found to be more localized within NAT and VNAT bases than within a HO basis for the optimal HO parameters, and, as anticipated, a core-valence (tensor product) structure emerges from the full six-body calculation of 6He. The two-nucleon mutual information shows that the VNAT basis, which typically exhibits good convergence properties, effectively decouples the active and inactive spaces. We conclude that measures of one- and two-nucleon entanglement are useful in analyzing the structure of nuclear wave functions, in particular the efficacy of basis states, and may provide useful metrics toward developing more efficient schemes for ab initio computations of the structure and reactions of nuclei, and quantum many-body systems more generally.
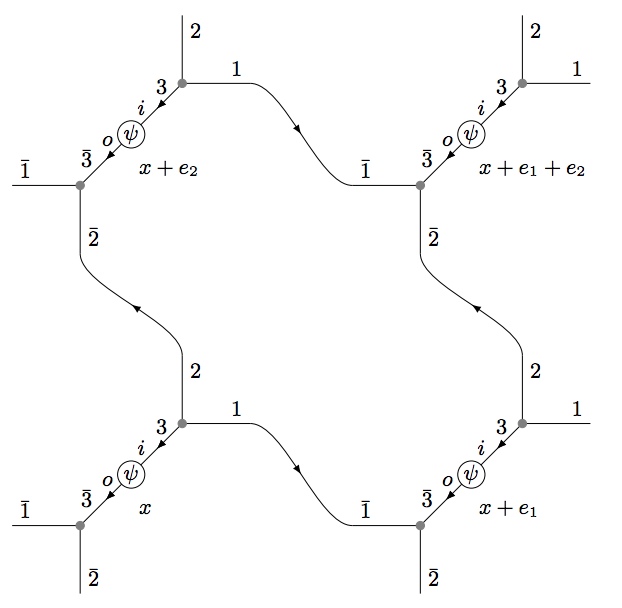
Loop, String, and Hadron Dynamics in SU(2) Hamiltonian Lattice Gauge Theories
The question of how to efficiently formulate Hamiltonian gauge theories is experiencing renewed interest due to advances in building quantum simulation platforms. We introduce a reformulation of an SU(2) Hamiltonian lattice gauge theory—a loop-string-hadron (LSH) formulation—that describes dynamics directly in terms of its loop, string, and hadron degrees of freedom, while alleviating several disadvantages of quantum-simulating the Kogut-Susskind formulation. This LSH formulation transcends the local loop formulation of d+1-dimensional lattice gauge theories by incorporating staggered quarks, furnishing the algebra of gauge-singlet operators, and being used to reconstruct dynamics between states that have Gauss’s law built in to them. LSH operators are then factored into products of “normalized” ladder operators and diagonal matrices, priming them for classical or quantum information processing. Self-contained expressions of the Hamiltonian are given up to d=3. The LSH formalism uses makes little use of structures specific to SU(2) and its conceptual clarity makes it an attractive approach to apply to other non-Abelian groups like SU(3).
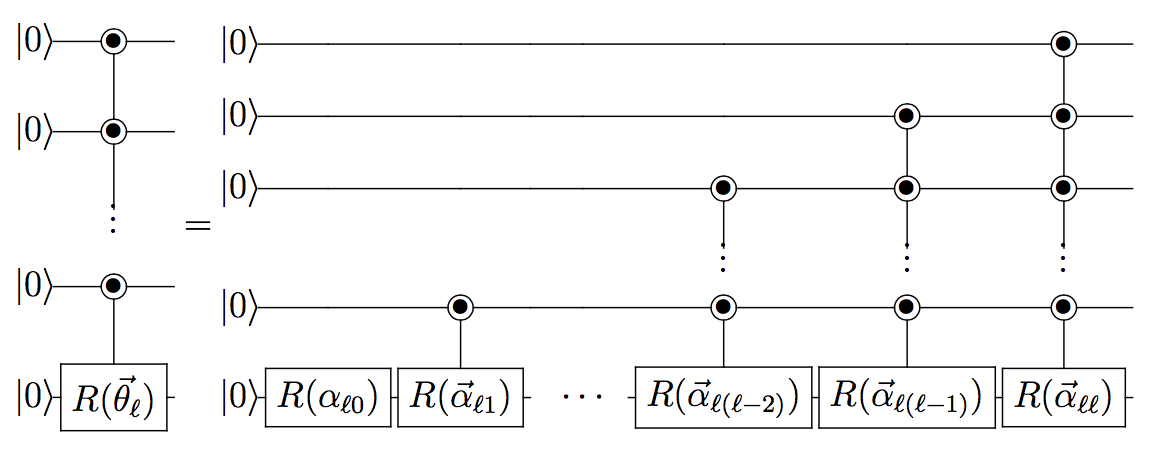
Systematically Localizable Operators for Quantum Simulations of Quantum Field Theories
Correlations and measures of entanglement in ground state wavefunctions of relativistic quantum field theories are spatially localized over length scales set by the mass of the lightest particle. We utilize this localization to design digital quantum circuits for preparing the ground states of free lattice scalar quantum field theories. Controlled rotations that are exponentially localized in their position-space extent are found to provide exponentially convergent wavefunction fidelity. These angles scale with the classical two-point correlation function, as opposed to the more localized mutual information or the hyper-localized negativity. We anticipate that further investigations will uncover quantum circuit designs with controlled rotations dictated by the measures of entanglement. This work is expected to impact quantum simulations of systems of importance to nuclear physics, high-energy physics, and basic energy sciences research.
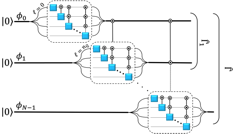
Fixed-Point Quantum Circuits for Quantum Field Theories
Renormalization group ideas and effective operators are used to efficiently determine localized unitaries for preparing the ground states of non-interacting scalar field theories on digital quantum devices. With these methods, classically computed ground states in a small spatial volume can be used to determine operators for preparing the ground state in a beyond-classical quantum register, even for interacting scalar field theories. Due to the exponential decay of correlation functions and the double exponential suppression of digitization artifacts, the derived quantum circuits are expected to be relevant already for near-term quantum devices.
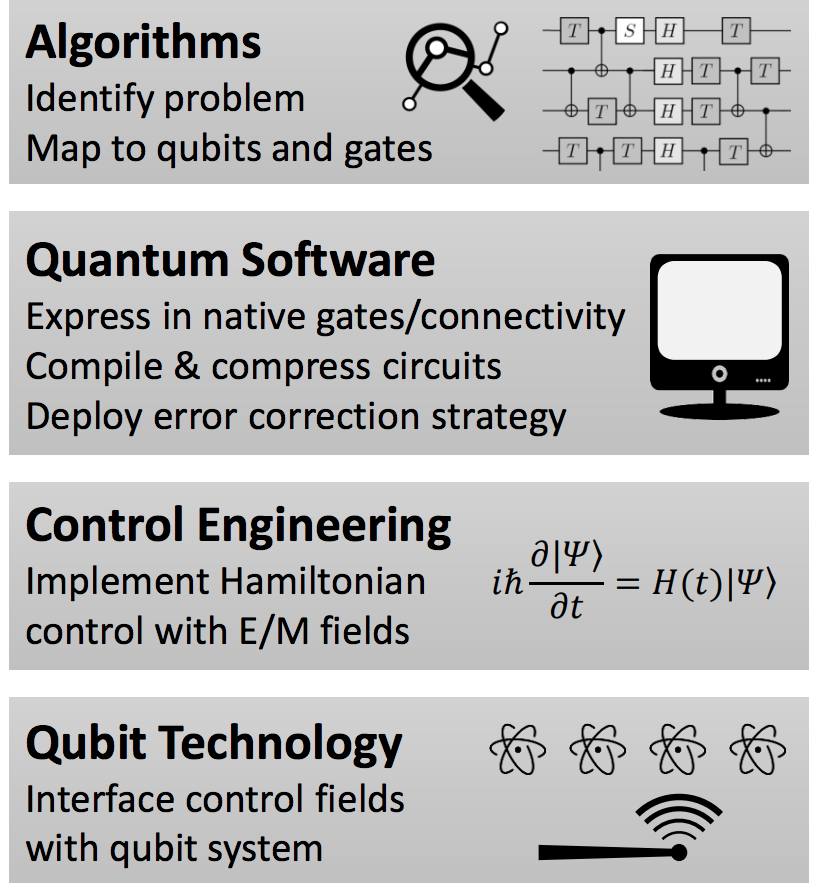
Quantum Computer Systems for Scientific Discovery
The great promise of quantum computers comes with the dual challenges of building them and finding their useful applications. We argue that these two challenges should be considered together, by co-designing full-stack quantum computer systems along with their applications in order to hasten their development and potential for scientific discovery. In this context, we identify scientific and community needs, opportunities, a sampling of a few use case studies, and significant challenges for the development of quantum computers for science over the next 2–10 years. This document is written by a community of university, national laboratory, and industrial researchers in the field of Quantum Information Science and Technology, and is based on a summary from a U.S. National Science Foundation workshop on Quantum Computing held on October 21–22, 2019 in Alexandria, VA.