IQuS Publications
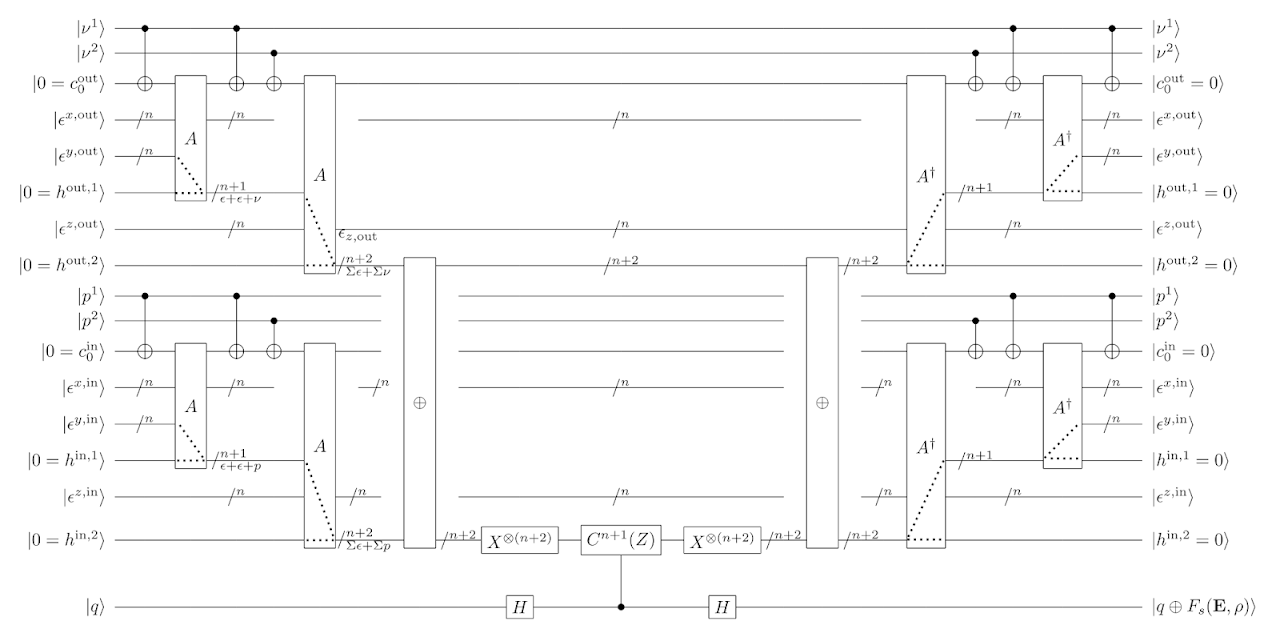
Oracles for Gauss’s Law on Digital Quantum Computers
Formulating a lattice gauge theory using only physical degrees of freedom generically leads to non-local interactions. A local Hamiltonian is desirable for quantum simulation, and this is possible by treating the Hilbert space as a subspace of a much larger Hilbert space in which Gauss’s law is not automatic. Digital quantum simulations of this local formulation will wander into unphysical sectors due to errors from Trotterization or from quantum noise. In this work, oracles are constructed that use local Gauss law constraints to projectively distinguish physical and unphysical wave functions in Abelian lattice gauge theories. Such oracles can be used to detect errors that break Gauss’s law.
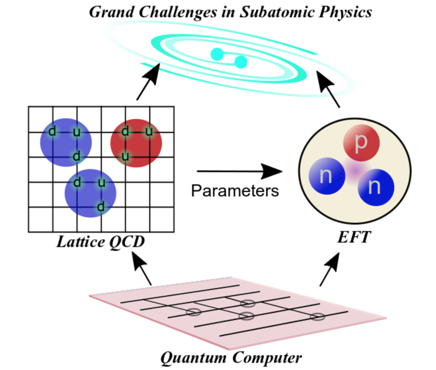
Simulations of Subatomic Many-Body Physics on a Quantum Frequency Processor
Simulating complex many-body quantum phenomena is a major scientific impetus behind the development of quantum computing, and a range of technologies are being explored to address such systems. We present the results of the largest photonics-based simulation to date, applied in the context of subatomic physics. Using an all-optical quantum frequency processor, the ground-state energies of light nuclei including the triton (3H), 3He, and the alpha particle (4He) are computed. Complementing these calculations and utilizing a 68-dimensional Hilbert space, our photonic simulator is used to perform sub-nucleon calculations of the two-body and three-body forces between heavy mesons in the Schwinger model. This work is a first step in simulating subatomic many-body physics on quantum frequency processors—augmenting classical computations that bridge scales from quarks to nuclei.[Image (left) was created by Pavel Lougovski.]
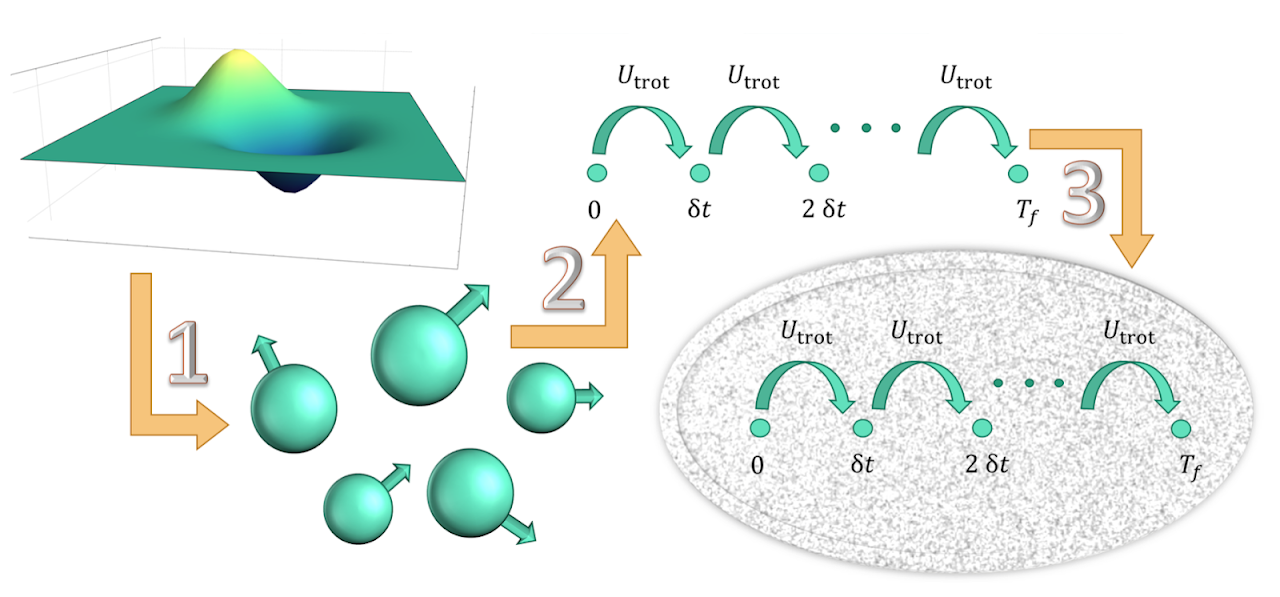
Digitization of Scalar Fields for NISQ-Era Quantum Computing
Qubit, operator and gate resources required for the digitization of lattice λϕ4 scalar field theories onto quantum computers in the NISQ era are considered, building upon the foundational work by Jordan, Lee and Preskill. The Nyquist-Shannon sampling theorem, introduced in this context by Macridin, Spetzouris, Amundson and Harnik building on the work of Somma, provides a guide with which to evaluate the efficacy of two field-space bases, the eigenstates of the field operator, as used by Jordan, Lee and Preskill, and eigenstates of a harmonic oscillator, to describe 0+1- and 1+1-dimensional scalar field theory. We show how techniques associated with improved actions, which are heavily utilized in Lattice QCD calculations to systematically reduce lattice-spacing artifacts, can be used to reduce the impact of the field digitization in λϕ4, but are found to be inferior to a complete digitization-improvement of the Hamiltonian using a Quantum Fourier Transform. When the Nyquist-Shannon sampling theorem is satisfied, digitization errors scale as |log|log|ϵdig|||∼nQ (number of qubits describing the field at a given spatial site) for the low-lying states, leaving the familiar power-law lattice-spacing and finite-volume effects that scale as |log|ϵlatt||∼NQ (total number of qubits in the simulation). We find that fewer than nQ=10 qubits per spatial lattice site are sufficient to reduce digitization errors below noise levels expected in NISQ-era quantum devices for both localized and delocalized field-space wavefunctions. For localized wavefunctions, nQ=4 qubits are likely to be sufficient for calculations requiring modest precision.
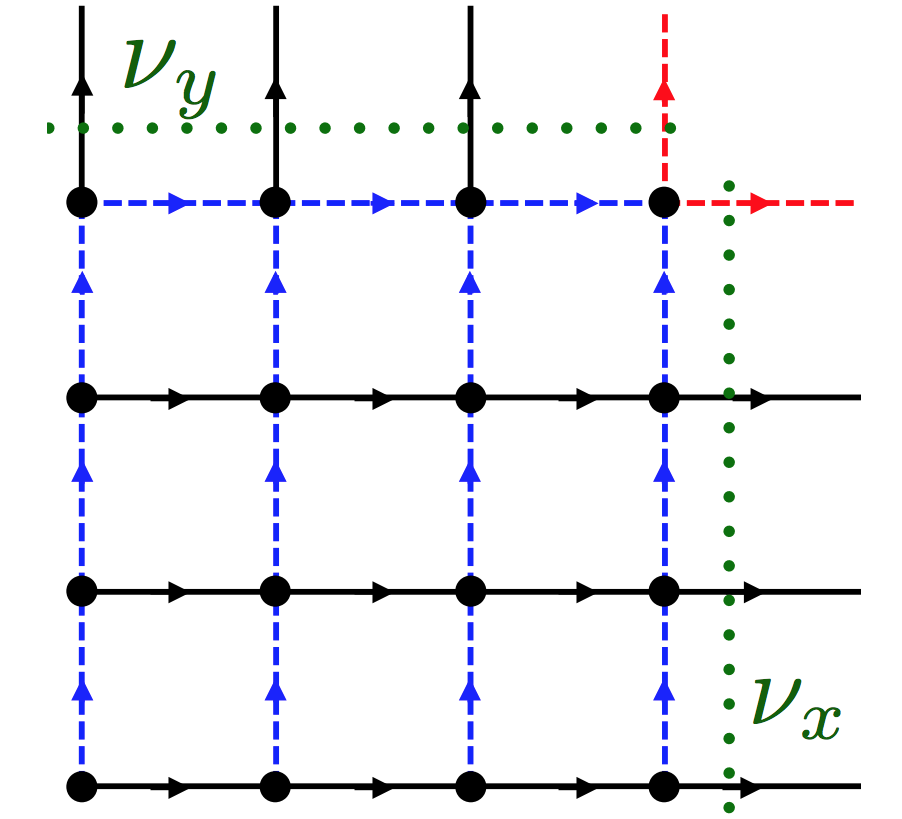
Gauss’s Law, Duality, and the Hamiltonian Formulation of U(1) Lattice Gauge Theory
Quantum computers have the potential to explore the vast Hilbert space of entangled states that play an important role in the behavior of strongly interacting matter. This opportunity motivates reconsidering the Hamiltonian formulation of gauge theories, with a suitable truncation scheme to render the Hilbert space finite-dimensional. Conventional formulations lead to a Hilbert space largely spanned by unphysical states; given the current inability to perform large scale quantum computations, we examine here how one might restrict wave function evolution entirely or mostly to the physical subspace. We consider such constructions for the simplest of these theories containing dynamical gauge bosons—U(1) lattice gauge theory without matter in d=2,3 spatial dimensions—and find that electric-magnetic duality naturally plays an important role. We conclude that this approach is likely to significantly reduce computational overhead in d=2 by a reduction of variables and by allowing one to regulate magnetic fluctuations instead of electric. The former advantage does not exist in d=3, but the latter might be important for asymptotically-free gauge theories.
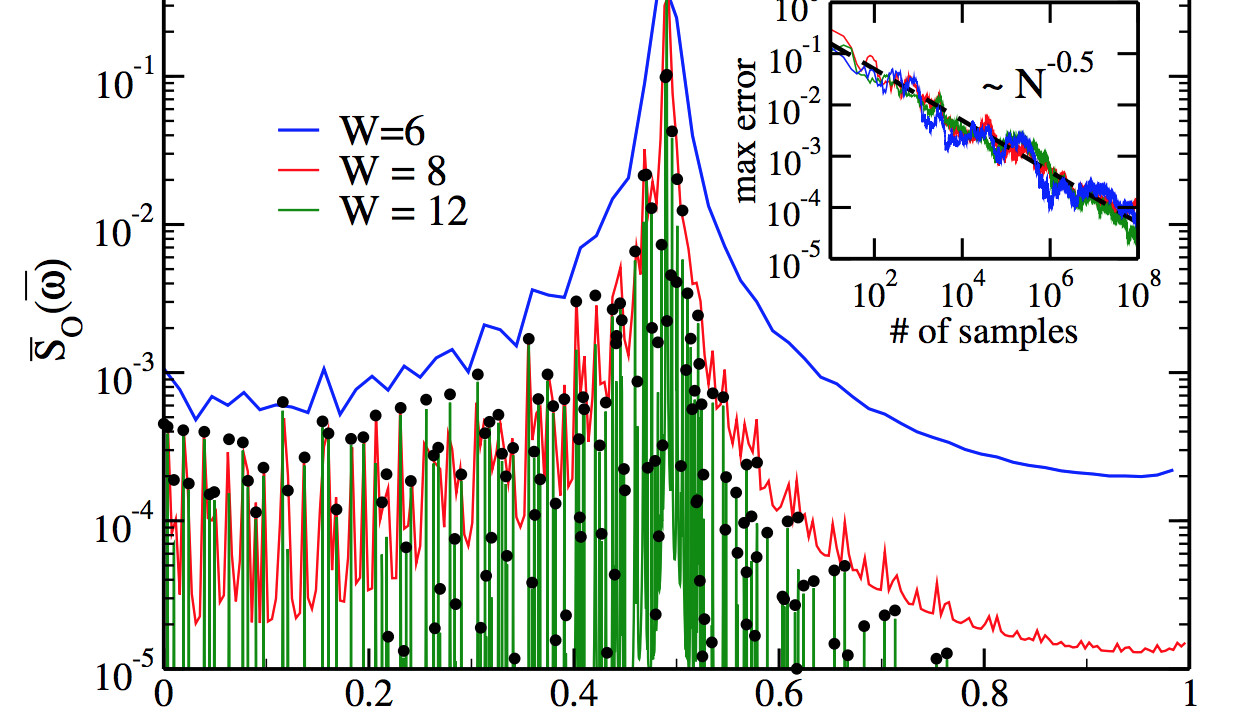
Linear Response on a Quantum Computer
The dynamic linear response of a quantum system is critical for understanding both the structure and dynamics of strongly-interacting quantum systems, including neutron scattering from materials, photon and electron scattering from atomic systems, and electron and neutrino scattering by nuclei. We present a general algorithm for quantum computers to calculate the dynamic linear response function with controlled errors and to obtain information about specific final states that can be directly compared to experimental observations.
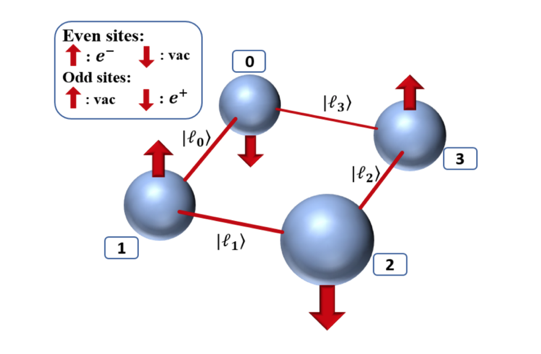
Quantum-classical computation of Schwinger model dynamics using quantum computers
We present a quantum-classical algorithm to study the dynamics of the two-spatial-site Schwinger model on IBM’s quantum computers. Using rotational symmetries, total charge, and parity, the number of qubits needed to perform computation is reduced by a factor of ∼5, removing exponentially-large unphysical sectors from the Hilbert space. Our work opens an avenue for exploration of other lattice quantum field theories, such as quantum chromodynamics, where classical computation is used to find symmetry sectors in which the quantum computer evaluates the dynamics of quantum fluctuations.
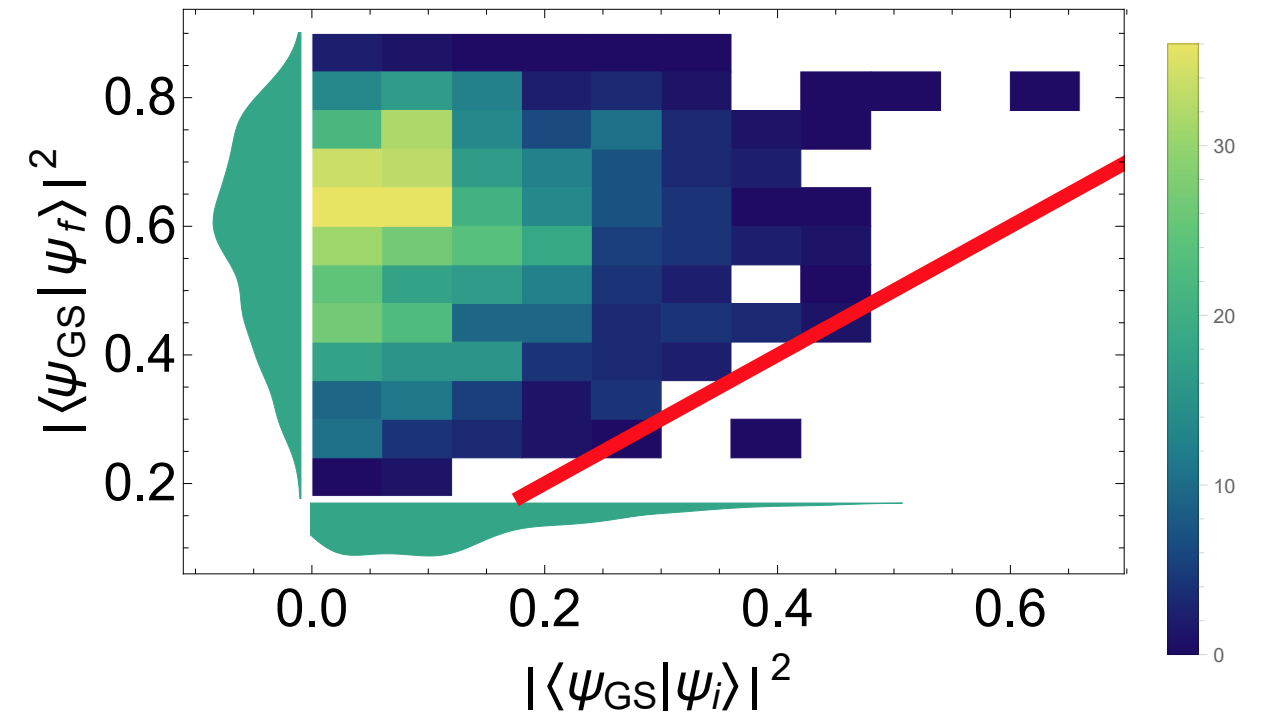
Ground States via Spectral Combing on a Quantum Computer
A new method is proposed for determining the ground state wave function of a quantum many-body system on a quantum computer, without requiring an initial trial wave function that has good overlap with the true ground state. The technique of Spectral Combing involves entangling an arbitrary initial wave function with a set of auxiliary qubits governed by a time dependent Hamiltonian, resonantly transferring energy out of the initial state through a plethora of avoided level crossings into the auxiliary system. The number of avoided level crossings grows exponentially with the number of qubits required to represent the Hamiltonian, so that the efficiency of the algorithm does not rely on any particular energy gap being large. We give an explicit construction of the quantum gates required for the realization of this procedure and explore the results of classical simulations of the algorithm on a small quantum computer with up to 8 qubits. We show that for certain systems and comparable results, Spectral Combing requires fewer quantum gates to realize than the Quantum Adiabatic Algorithm.