IQuS Publications
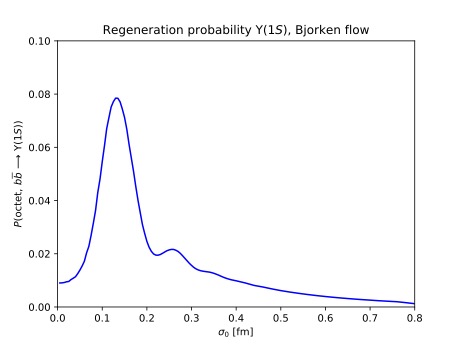
Quarkonium transport in weakly and strongly coupled plasmas
We report on progress in the nonperturbative understanding of quarkonium dynamics inside a thermal plasma. The time evolution of small-size quarkonium is governed by two-point correlation functions of chromoelectric fields dressed with an adjoint Wilson line, known in this context as generalized gluon distributions (GGDs). The GGDs have been calculated in both weakly and strongly coupled plasmas by using perturbative and holographic methods. Strikingly, the results of our calculations for a strongly coupled plasma indicate that the quarkonium dissociation and recombination rates vanish in the transport descriptions that assume quarkonium undergoes Markovian dynamics. However, this does not imply that the dynamics is trivial. As a starting point to explore the phenomenological consequences of the result at strong coupling, we show a calculation of the $\Upsilon(1S)$ formation probability in time-dependent perturbation theory. This is a first step towards the development of a transport formalism that includes non-Markovian effects, which, depending on how close the as of yet undetermined nonperturbative QCD result of the GGDs is to the strongly coupled $\mathcal{N}=4$ SYM result, could very well dominate over the Markovian ones in quark-gluon plasma produced at RHIC and the LHC.
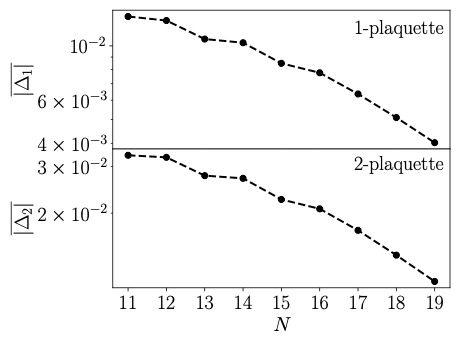
Testing Eigenstate Thermalization Hypothesis for Non-Abelian Gauge Theories
We report on progress in full quantum understanding of thermalization in non-Abelian gauge theories. Specifically, we test the eigenstate thermalization hypothesis for (2+1)-dimensional SU(2) lattice gauge theory.
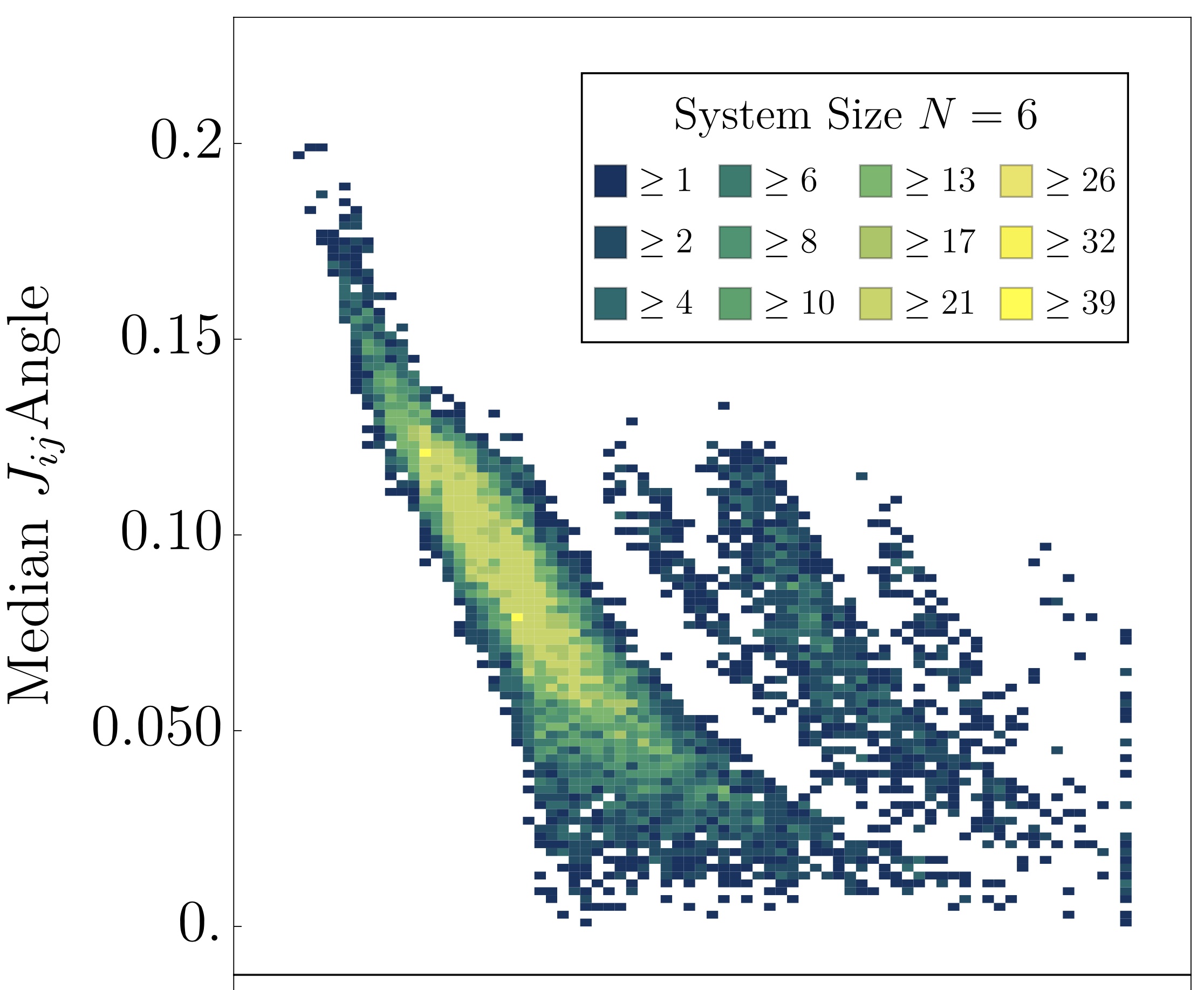
Time Scales in Many-Body Fast Neutrino Flavor Conversion
Time scales associated with many-body fast neutrino flavor conversions in core-collapse supernova are explored in the context of an effective two-flavor “light-bulb” model. We present a preliminary study of time scales obtained from linear stability analyses and from distributions of Loschmidt echo crossing times (intimately connected to dynamical phase transitions) determined by time evolution with the exact many-body Hamiltonian. Starting from a tensor-product initial state describing systems of N neutrinos, with N/2 electron-type and N/2 heavy-type, with uniform distributions of axially-symmetric directions, the Loschmidt echo crossing times are found to exhibit two distinct time scales that are exponentially separated. The second peak structure at longer times, effectively absent for N=4, develops with increasing N. When re-scaled in terms of log t, the distributions are found to become increasingly well described by the sum of two stable distributions. The distribution of Loschmidt echo crossing times differs somewhat from the results of the linear stability analysis, which exhibits a peak at finite frequency and a second peak at zero frequency. The exact analysis suggests that the zero-frequency instability manifests itself as a modest flavor-conversion time scale.
This work was supported in part by U.S. Department of Energy, Office of Science, Office of Nuclear Physics, InQubator for Quantum Simulation (IQuS) under Award Number DOE (NP) Award DE-SC0020970 via the program on Quantum Horizons: QIS Research and Innovation for Nuclear Science. This work is also supported, in part, through the Department of Physics and the College of Arts and Sciences at the University of Washington.
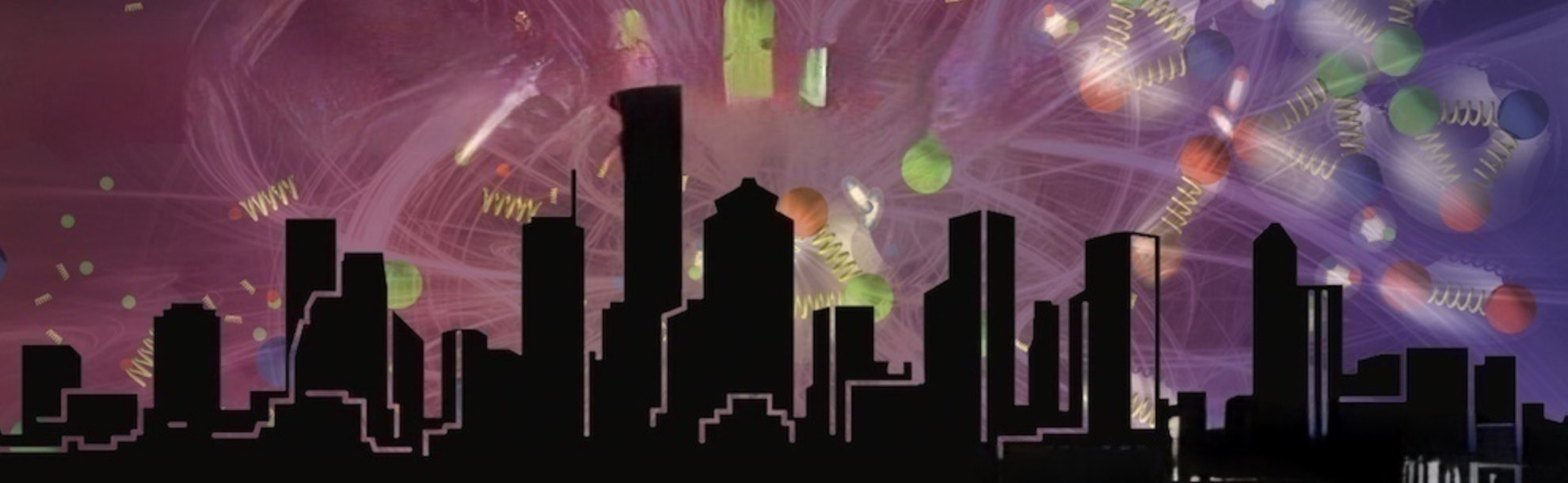
Quantum Computing for Nuclear Physics
Future quantum computers are anticipated to be able to perform simulations of quantum many-body systems and quantum field theories that lie beyond the capabilities of classical computation. This will lead to new insights and predictions for systems ranging from dense non equilibrium matter, to low-energy nuclear structure and reactions, to high energy collisions. I present an overview of digital quantum simulations in nuclear physics, with select examples relevant for studies of quark matter.
Conference proceedings for Quark Matter 2023, 3–9 Sept 2023, Houston, Texas, USA.
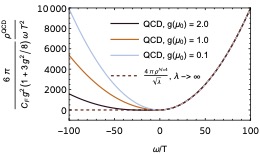
Generalized Gluon Distribution for Quarkonium Dynamics in Strongly Coupled N=4 Yang-Mills Theory
We study the generalized gluon distribution that governs the dynamics of quarkonium inside a non-Abelian thermal plasma characterizing its dissociation and recombination rates. This gluon distribution can be written in terms of a correlation function of two chromoelectric fields connected by an adjoint Wilson line. We formulate and calculate this object in N=4 supersymmetric Yang-Mills theory at strong coupling using the AdS/CFT correspondence, allowing for a nonzero center-of-mass velocity v of the heavy quark pair relative to the medium. The effect of a moving medium on the dynamics of the heavy quark pair is described by the simple substitution T with Sqrt[gamma] T, in agreement with previous calculations of other observables at strong coupling, where T is the temperature of the plasma in its rest frame, and gamma = (1 – v^2)^{-1/2} is the Lorentz boost factor. Such a velocity dependence can be important when the quarkonium momentum is larger than its mass. Contrary to general expectations for open quantum systems weakly coupled with large thermal environments, the contributions to the transition rates that are usually thought of as the leading ones in Markovian descriptions vanish in this strongly coupled plasma. This calls for new theoretical developments to assess the effects of strongly coupled non-Abelian plasmas on in-medium quarkonium dynamics. Finally, we compare our results with those from weakly coupled QCD, and find that the QCD result moves toward the N=4 strongly coupled result as the coupling constant is increased within the regime of applicability of perturbation theory. This behavior makes it even more pressing to develop a non-Markovian description of quarkonium in-medium dynamics.
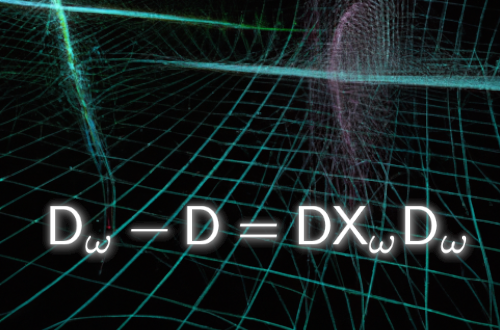
Generalized Ginsparg-Wilson relations
We give a general derivation of Ginsparg-Wilson relations for both Dirac and Majorana fermions in any dimension. These relations encode continuous and discrete chiral, parity and time reversal anomalies and will apply to the various classes of free fermion topological insulators and superconductors (in the framework of a relativistic quantum field theory in Euclidian spacetime). We show how to formulate the exact symmetries of the lattice action and the relevant index theorems for the anomalies.
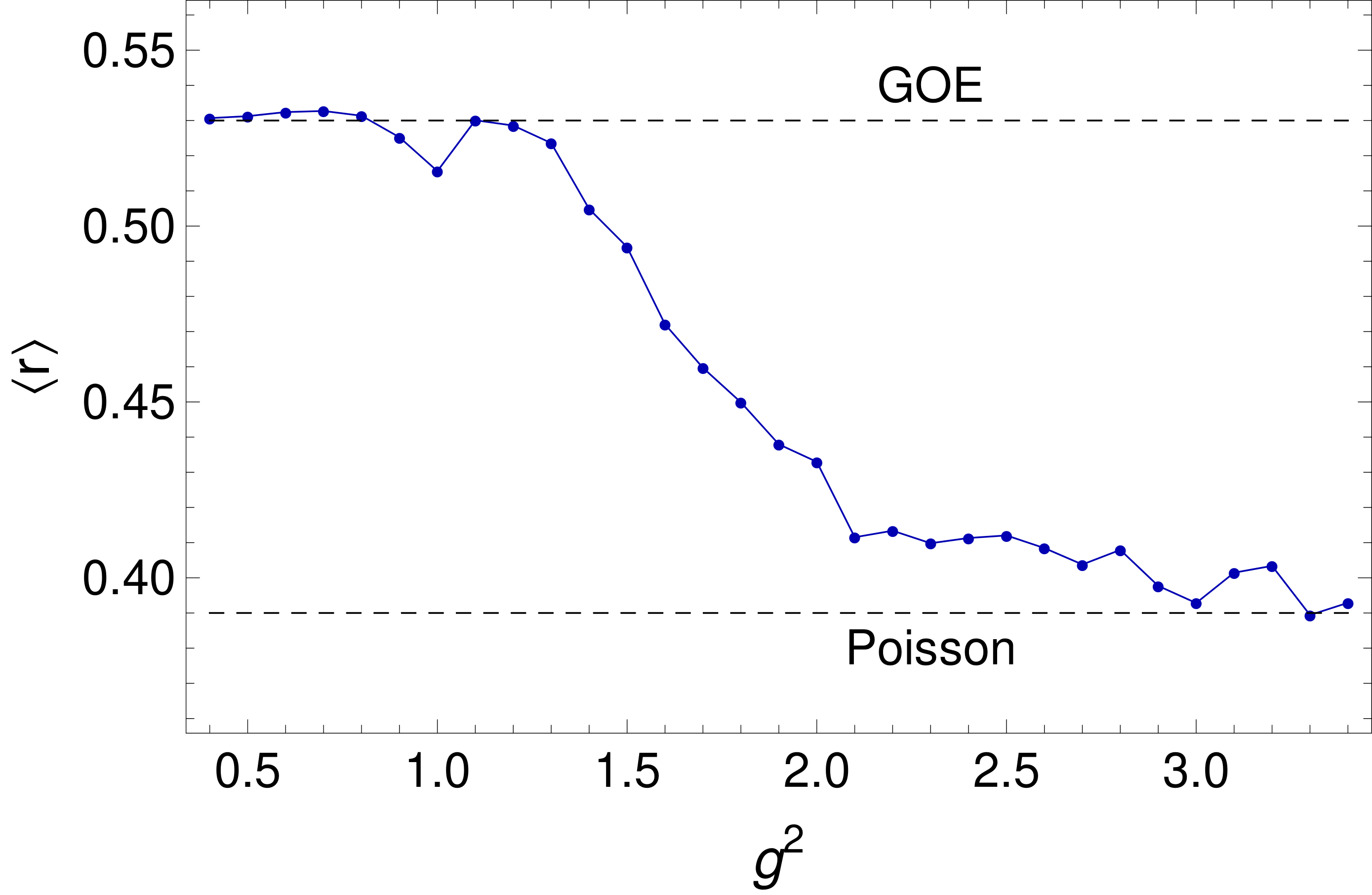
Eigenstate Thermalization in 2+1 dimensional SU(2) Lattice Gauge Theory
We present preliminary numerical evidence for the hypothesis that the Hamiltonian SU(2) gauge theory discretized on a lattice obeys the Eigenstate Thermalization Hypothesis (ETH). To do so we study three approximations: (a) a linear plaquette chain in a reduced Hilbert space limiting the electric field basis to j=0,1/2 , (b) a two-dimensional honeycomb lattice with periodic or closed boundary condition and the same Hilbert space constraint, and (c) a chain of only three plaquettes but such a sufficiently large electric field Hilbert space (j <= 7/2) that convergence of all energy eigenvalues in the analyzed energy window is observed. While an unconstrained Hilbert space is required to reach the continuum limit of SU(2) gauge theory, numerical resource constraints do not permit us to realize this requirement for all values of the coupling constant and large lattices. In each of the three studied cases we check first for RMT behavior and then analyse the diagonal as well as the off-diagonal matrix elements between energy eigenstates for a few operators. Within current uncertainties all results for (b) and (c) agree with ETH predictions while for case (a) deviations are found to be large for one of the analyzed observables. To unambiguously establish ETH behavior and determine for which class of operators it applies, an extension of our investigations is necessary.
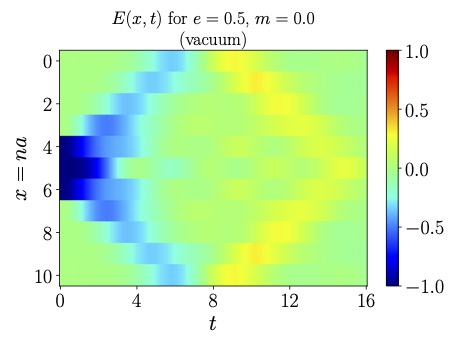
Liouvillian Dynamics of the Open Schwinger Model: String Breaking and Kinetic Dissipation in a Thermal Medium
Understanding the dynamics of bound state formation is one of the fundamental questions in confining quantum field theories such as Quantum Chromodynamics (QCD). One hadronization mechanism that has garnered significant attention is the breaking of a string initially connecting a fermion and an anti-fermion. Deepening our understanding of real-time string-breaking dynamics with simpler, lower dimensional models like the Schwinger model can improve our understanding of the hadronization process in QCD and other confining systems found in condensed matter and statistical systems. In this paper, we consider the string-breaking dynamics within the Schwinger model and investigate its modification inside a thermal medium, treating the Schwinger model as an open quantum system coupled to a thermal environment. Within the regime of weak coupling between the system and environment, the real-time evolution of the system can be described by a Lindblad evolution equation. We analyze the Liouvillian gaps of this Lindblad equation and the time dependence of the system’s von Neumann entropy. We observe that the late-time relaxation rate decreases as the environment correlation length increases. Moreover, when the environment correlation length is infinite, the system exhibits two steady states, one in each of the sectors with definite charge-conjugation-parity (CP) quantum numbers. For parameter regimes where an initial string breaks in vacuum, we observe a delay of the string breaking in the medium, due to kinetic dissipation effects. Conversely, in regimes where an initial string remains intact in vacuum time evolution, we observe string breaking (melting) in the thermal medium. We further discuss how the Liouvillian dynamics of the open Schwinger model can be simulated on quantum computers and provide an estimate of the associated Trotter errors.
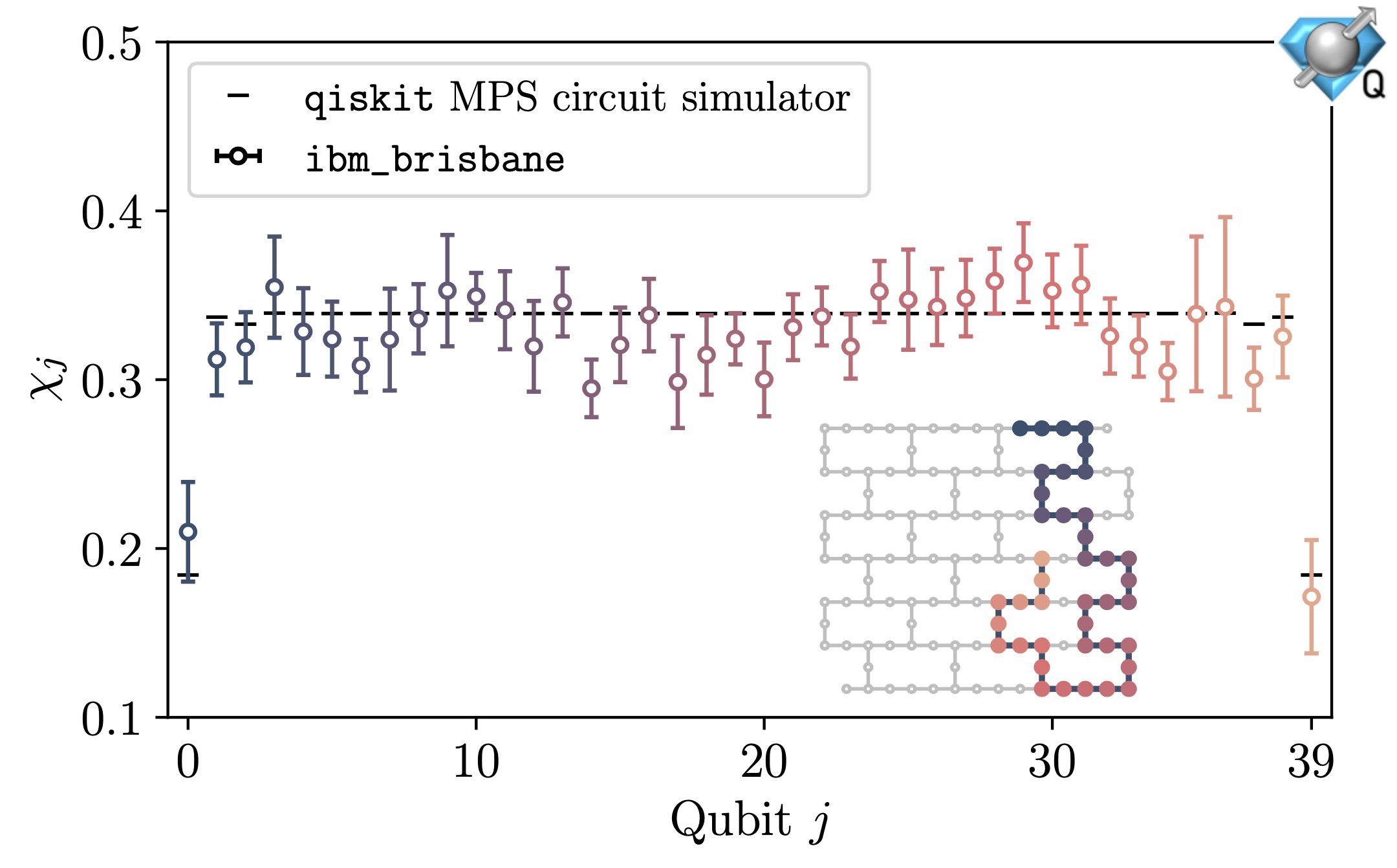
Scalable Circuits for Preparing Ground States on Digital Quantum Computers: The Schwinger Model Vacuum on 100 Qubits
The vacuum of the lattice Schwinger model is prepared on up to 100 qubits of IBM’s Eagle-processor quantum computers. A new algorithm to prepare the ground state of a gapped translationally-invariant system on a quantum computer is presented, which we call Scalable Circuits ADAPT-VQE (SC ADAPT-VQE). This algorithm uses the exponential decay of correlations between distant regions of the ground state, together with ADAPT-VQE, to construct quantum circuits for state preparation that can be scaled to arbitrarily large systems. SC-ADAPT-VQE is applied to the Schwinger model, and shown to be systematically improvable, with an accuracy that converges exponentially with circuit depth. Both the structure of the circuits and the deviations of prepared wavefunctions are found to become independent of the number of spatial sites, L. This allows for a controlled extrapolation of the circuits, determined using small or modest-sized systems, to arbitrarily large L. The circuits for the Schwinger model are determined on lattices up to L=14 (28 qubits) with the qiskit classical simulator, and subsequently scaled up to prepare the L=50 (100 qubits) vacuum on IBM’s 127 superconducting-qubit quantum computers ibm_brisbane and ibm_cusco . After applying an improved error-mitigation technique, which we call Operator Decoherence Renormalization, the chiral condensate and charge-charge correlators obtained from the quantum computers are found to be in good agreement with classical Matrix Product State simulations.
This work was supported, in part, by the U.S. Department of Energy grant DE-FG02-97ER-41014 (Farrell), by U.S. Department of Energy, Office of Science, Office of Nuclear Physics, InQubator for Quantum Simulation (IQuS) under Award Number DOE (NP) Award DE-SC0020970 via the program on Quantum Horizons: QIS Research and Innovation for Nuclear Science (Ciavarella, Farrell, Savage), and the Quantum Science Center (QSC), a National Quantum Information Science Research Center of the U.S. Department of Energy (DOE) (Illa). This work was also supported, in part, through the Department of Physics and the College of Arts and Sciences at the University of Washington.
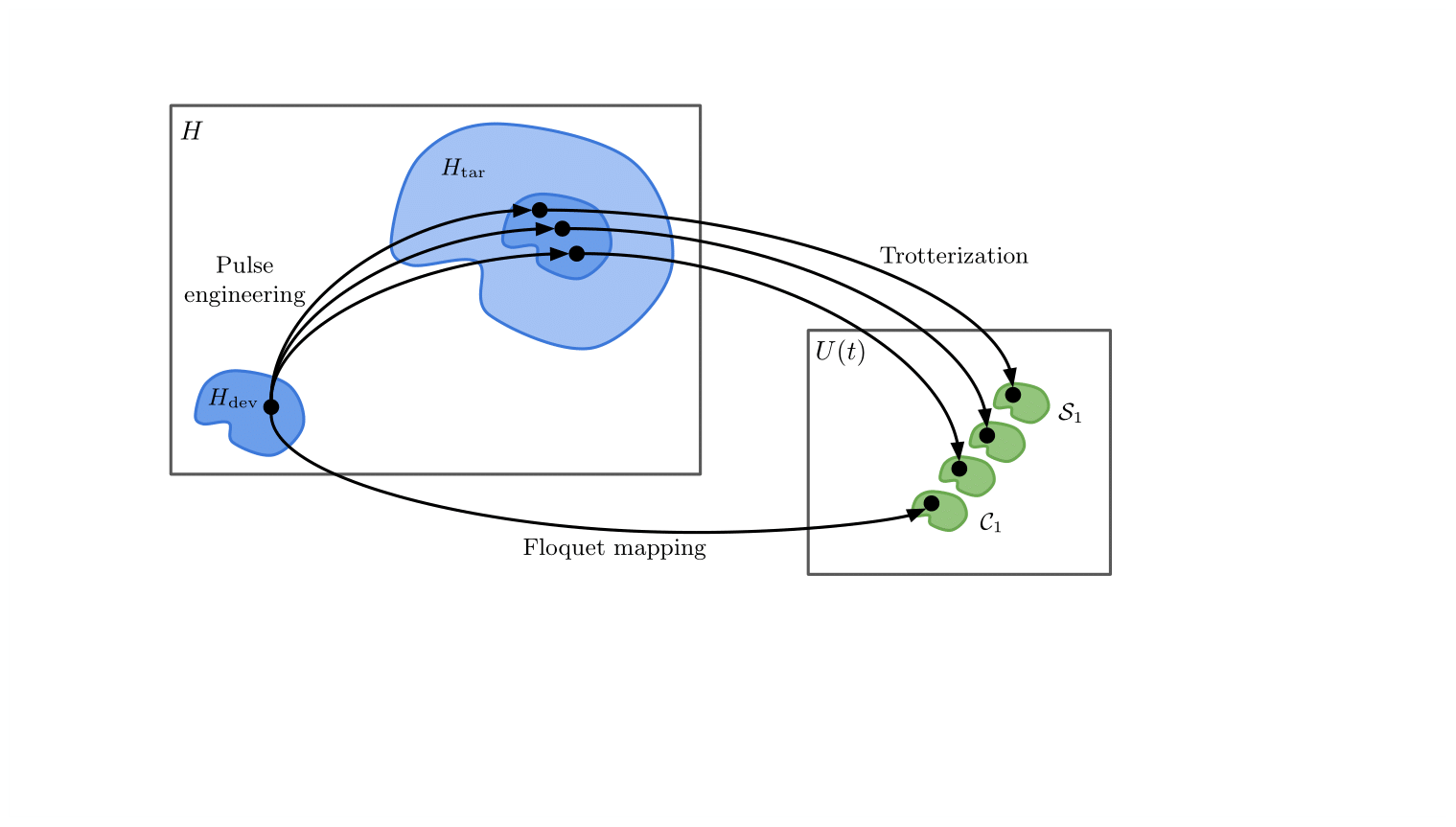
Optimization of Algorithmic Errors in Analog Quantum Simulations
Analog quantum simulation is emerging as a powerful tool for uncovering classically unreachable physics such as many-body real-time dynamics. We study a class of errors for approximate time evolution algorithms in Heisenberg-type systems on analog quantum devices described by the Ising Hamiltonian. A general framework for quantifying these errors is introduced and applied to several proposed time evolution methods, including Trotter-like methods and Floquet-engineered constant-field approaches. This analysis explains the interplay of different error sources arising from approximations to the propagator of a physical theory. The limitations placed on the accuracy of time evolution methods by current devices are discussed. Characterization of the error scaling provides a way to extend the presented Hamiltonian engineering methods to take advantage of forthcoming device capabilities.