IQuS Publications
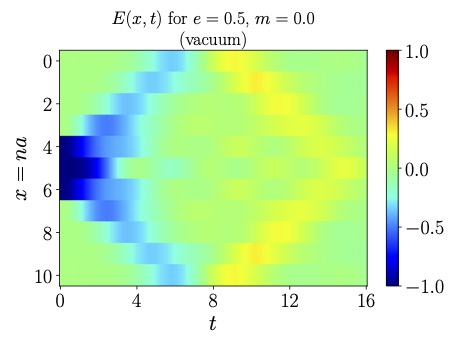
Liouvillian Dynamics of the Open Schwinger Model: String Breaking and Kinetic Dissipation in a Thermal Medium
Understanding the dynamics of bound state formation is one of the fundamental questions in confining quantum field theories such as Quantum Chromodynamics (QCD). One hadronization mechanism that has garnered significant attention is the breaking of a string initially connecting a fermion and an anti-fermion. Deepening our understanding of real-time string-breaking dynamics with simpler, lower dimensional models like the Schwinger model can improve our understanding of the hadronization process in QCD and other confining systems found in condensed matter and statistical systems. In this paper, we consider the string-breaking dynamics within the Schwinger model and investigate its modification inside a thermal medium, treating the Schwinger model as an open quantum system coupled to a thermal environment. Within the regime of weak coupling between the system and environment, the real-time evolution of the system can be described by a Lindblad evolution equation. We analyze the Liouvillian gaps of this Lindblad equation and the time dependence of the system’s von Neumann entropy. We observe that the late-time relaxation rate decreases as the environment correlation length increases. Moreover, when the environment correlation length is infinite, the system exhibits two steady states, one in each of the sectors with definite charge-conjugation-parity (CP) quantum numbers. For parameter regimes where an initial string breaks in vacuum, we observe a delay of the string breaking in the medium, due to kinetic dissipation effects. Conversely, in regimes where an initial string remains intact in vacuum time evolution, we observe string breaking (melting) in the thermal medium. We further discuss how the Liouvillian dynamics of the open Schwinger model can be simulated on quantum computers and provide an estimate of the associated Trotter errors.
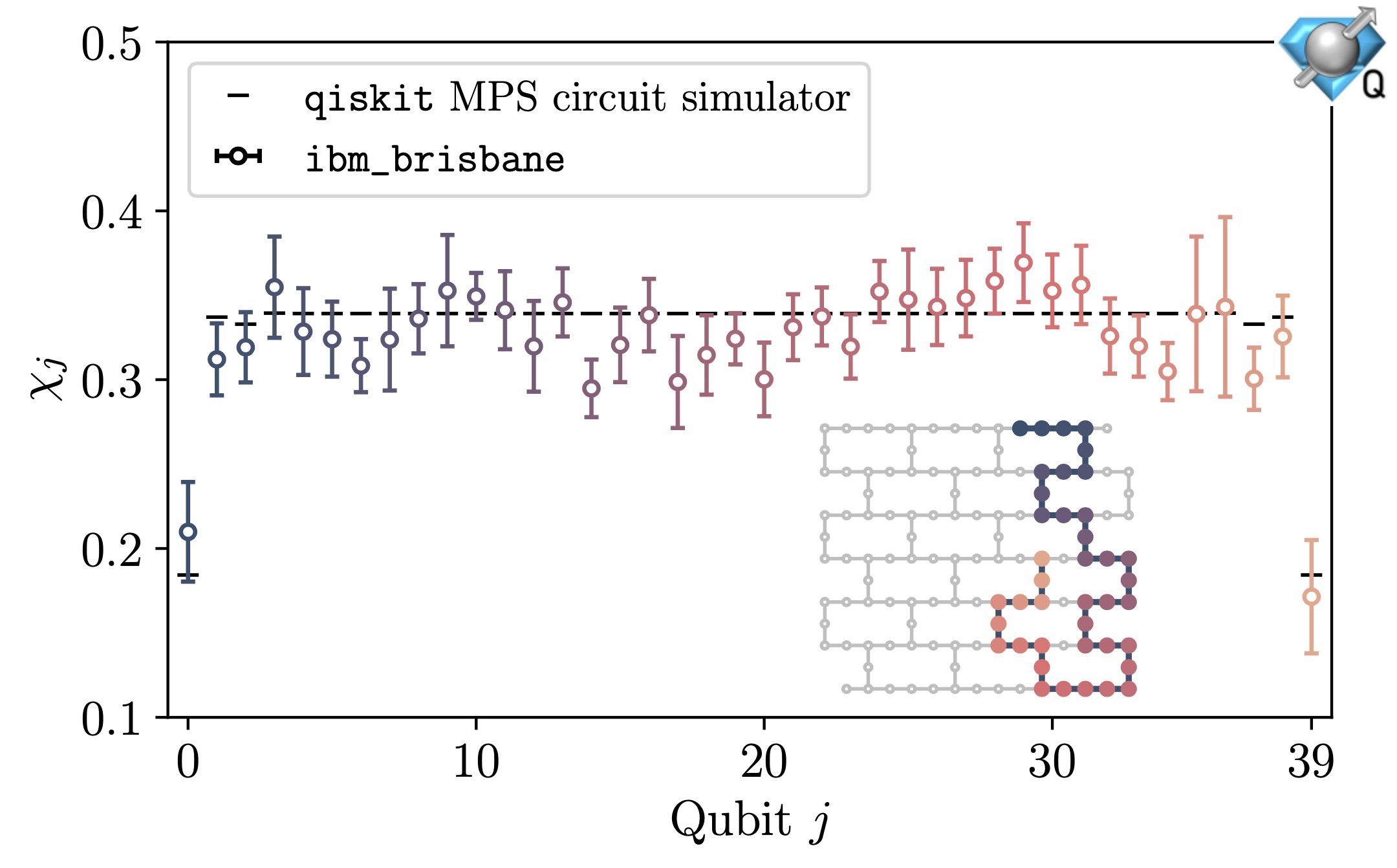
Scalable Circuits for Preparing Ground States on Digital Quantum Computers: The Schwinger Model Vacuum on 100 Qubits
The vacuum of the lattice Schwinger model is prepared on up to 100 qubits of IBM’s Eagle-processor quantum computers. A new algorithm to prepare the ground state of a gapped translationally-invariant system on a quantum computer is presented, which we call Scalable Circuits ADAPT-VQE (SC ADAPT-VQE). This algorithm uses the exponential decay of correlations between distant regions of the ground state, together with ADAPT-VQE, to construct quantum circuits for state preparation that can be scaled to arbitrarily large systems. SC-ADAPT-VQE is applied to the Schwinger model, and shown to be systematically improvable, with an accuracy that converges exponentially with circuit depth. Both the structure of the circuits and the deviations of prepared wavefunctions are found to become independent of the number of spatial sites, L. This allows for a controlled extrapolation of the circuits, determined using small or modest-sized systems, to arbitrarily large L. The circuits for the Schwinger model are determined on lattices up to L=14 (28 qubits) with the qiskit classical simulator, and subsequently scaled up to prepare the L=50 (100 qubits) vacuum on IBM’s 127 superconducting-qubit quantum computers ibm_brisbane and ibm_cusco . After applying an improved error-mitigation technique, which we call Operator Decoherence Renormalization, the chiral condensate and charge-charge correlators obtained from the quantum computers are found to be in good agreement with classical Matrix Product State simulations.
This work was supported, in part, by the U.S. Department of Energy grant DE-FG02-97ER-41014 (Farrell), by U.S. Department of Energy, Office of Science, Office of Nuclear Physics, InQubator for Quantum Simulation (IQuS) under Award Number DOE (NP) Award DE-SC0020970 via the program on Quantum Horizons: QIS Research and Innovation for Nuclear Science (Ciavarella, Farrell, Savage), and the Quantum Science Center (QSC), a National Quantum Information Science Research Center of the U.S. Department of Energy (DOE) (Illa). This work was also supported, in part, through the Department of Physics and the College of Arts and Sciences at the University of Washington.
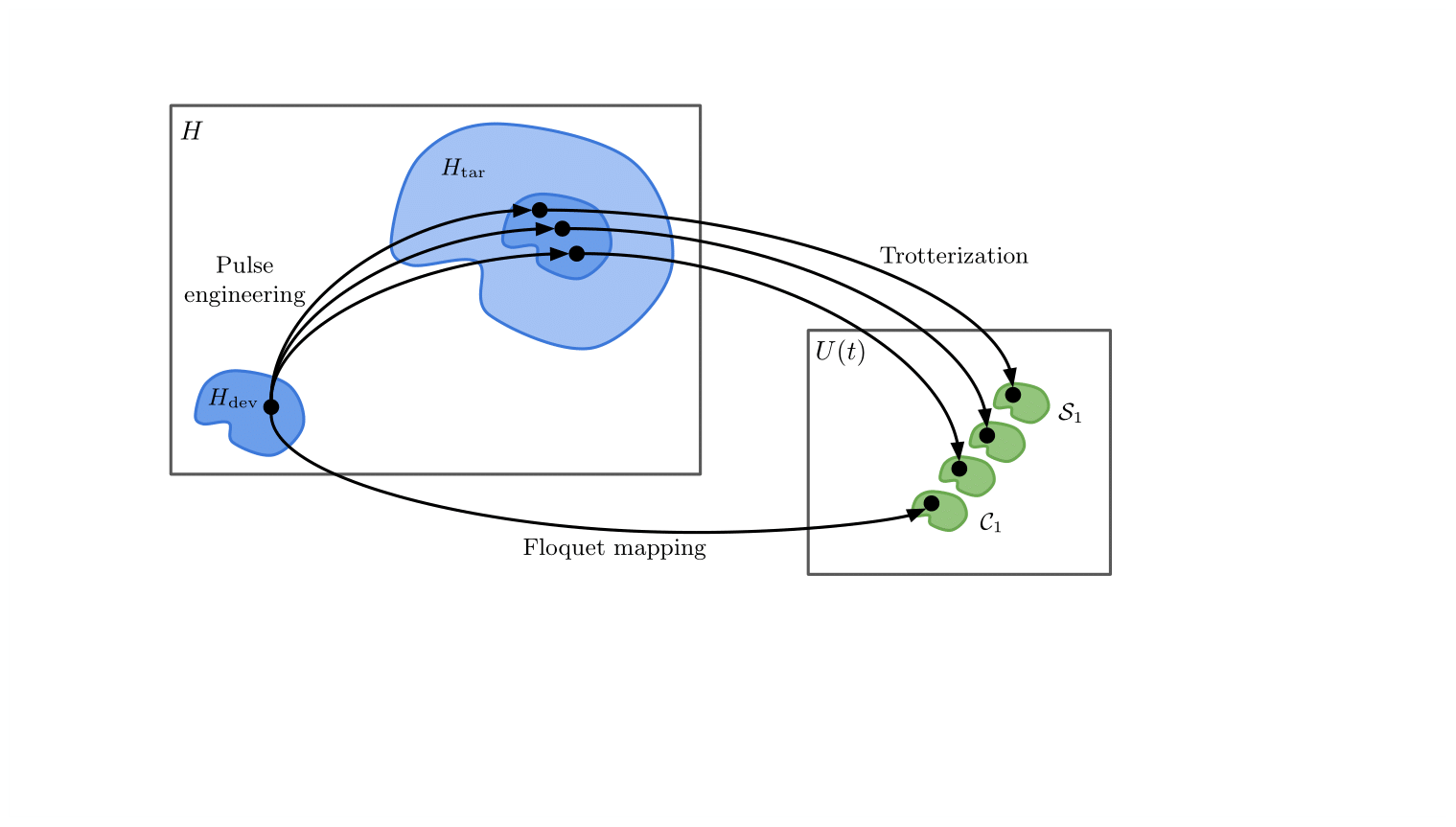
Optimization of Algorithmic Errors in Analog Quantum Simulations
Analog quantum simulation is emerging as a powerful tool for uncovering classically unreachable physics such as many-body real-time dynamics. We study a class of errors for approximate time evolution algorithms in Heisenberg-type systems on analog quantum devices described by the Ising Hamiltonian. A general framework for quantifying these errors is introduced and applied to several proposed time evolution methods, including Trotter-like methods and Floquet-engineered constant-field approaches. This analysis explains the interplay of different error sources arising from approximations to the propagator of a physical theory. The limitations placed on the accuracy of time evolution methods by current devices are discussed. Characterization of the error scaling provides a way to extend the presented Hamiltonian engineering methods to take advantage of forthcoming device capabilities.
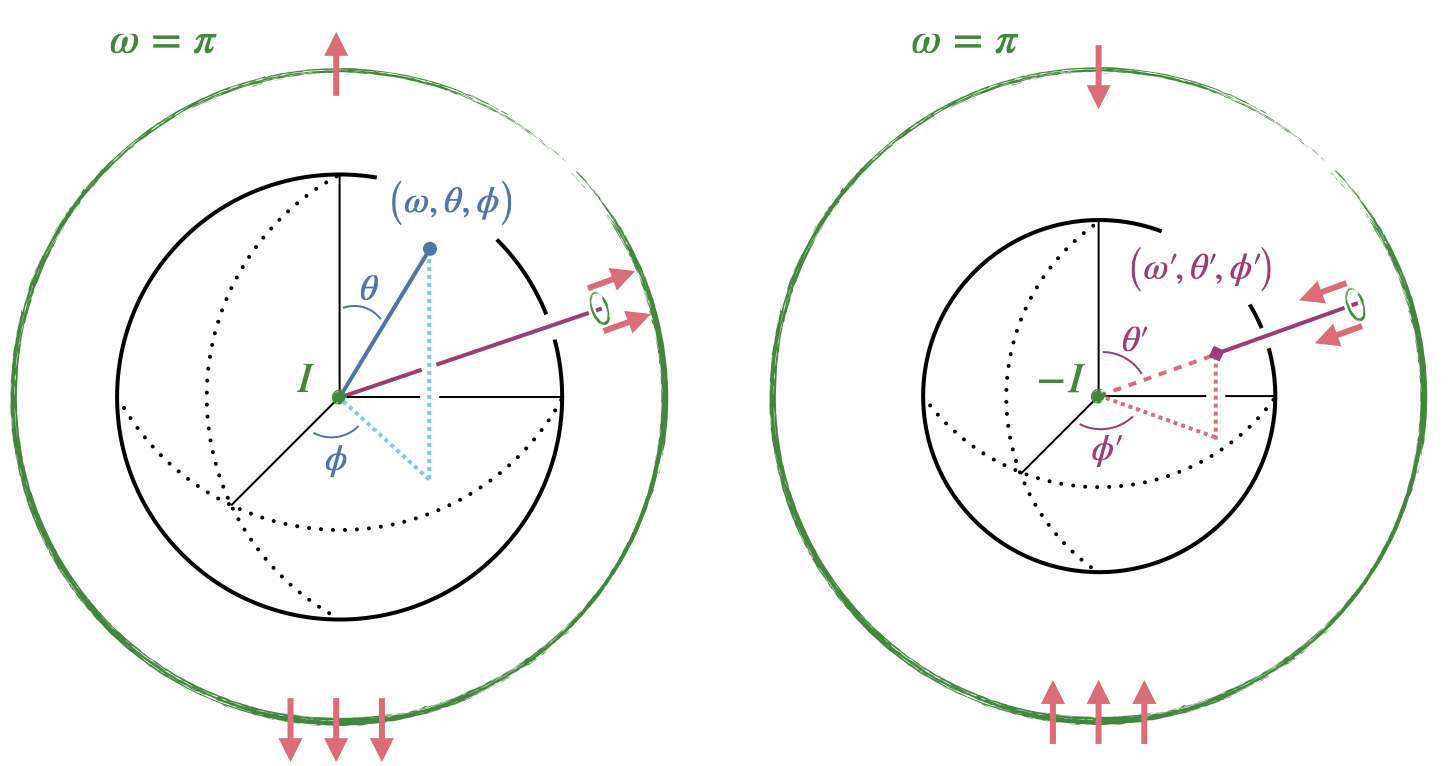
A new basis for Hamiltonian SU(2) simulations
Due to rapidly improving quantum computing hardware, Hamiltonian simulations of relativistic lattice field theories have seen a resurgence of attention. This computational tool requires turning the formally infinite-dimensional Hilbert space of the full theory into a finite-dimensional one. For gauge theories, a widely-used basis for the Hilbert space relies on the representations induced by the underlying gauge group, with a truncation that keeps only a set of the lowest dimensional representations. This works well at large bare gauge coupling, but becomes less efficient at small coupling, which is required for the continuum limit of the lattice theory. In this work, we develop a new basis suitable for the simulation of an SU(2) lattice gauge theory in the maximal tree gauge. In particular, we show how to perform a Hamiltonian truncation so that the eigenvalues of both the magnetic and electric gauge-fixed Hamiltonian are mostly preserved, which allows for this basis to be used at all values of the coupling. Little prior knowledge is assumed, so this may also be used as an introduction to the subject of Hamiltonian formulations of lattice gauge theories.
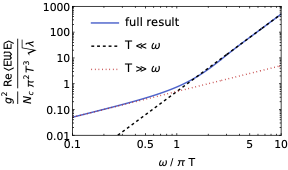
Quarkonium transport in strongly coupled plasmas
Suppression of open heavy flavors and quarkonia in heavy-ion collisions is among the most informative probes of the quark-gluon plasma. Interpreting the full wealth of data obtained from the collision events requires a precise understanding of the evolution of heavy quarks and quarkonia as they propagate through the nearly thermal and strongly coupled plasma. Systematic theoretical studies of quarkonium time evolution in the QGP in the regime where the temperature of the QGP is much smaller than the inverse of quarkonium size have only been carried out in the past few years.
Such calculations require the evaluation of a gauge-invariant correlator of chromoelectric fields dressed with Wilson lines, which is similar to, but different from, the correlation used to define the well-known heavy quark diffusion coefficient. The origin of this difference has been explained previously. Here we show a calculation of the analogous correlator in strongly coupled N=4 SYM using the AdS/CFT correspondence at a finite temperature. While it resembles the open heavy quark case, it has some crucial differences that highlight the relevance of quantum color correlations. We will also discuss the results for the quarkonium transport coefficients obtained from this correlator, thereby establishing the first analytic results at strong coupling in this context.
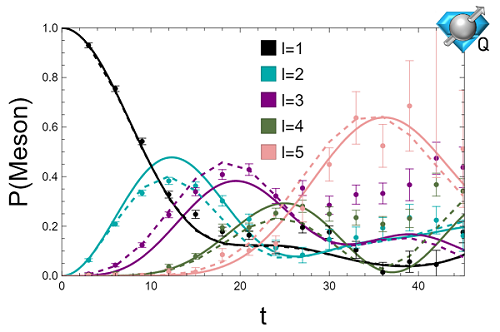
Quantum Simulation of Lattice QCD with Improved Hamiltonians
Quantum simulations of lattice gauge theories are anticipated to directly probe the real time dynamics of QCD, but scale unfavorably with the required truncation of the gauge fields. Improved Hamiltonians are derived to correct for the effects of gauge field truncations on the SU(3) Kogut-Susskind Hamiltonian. It is shown in 1+1D that this enables low chromo-electric field truncations to quantitatively reproduce features of the untruncated theory over a range of couplings and quark masses. In 3+1D, an improved Hamiltonian is derived for lattice QCD with staggered massless fermions. It is shown in the strong coupling limit that the spectrum qualitatively reproduces aspects of two flavor QCD and simulations of a small system are performed on IBM’s Perth quantum processor.
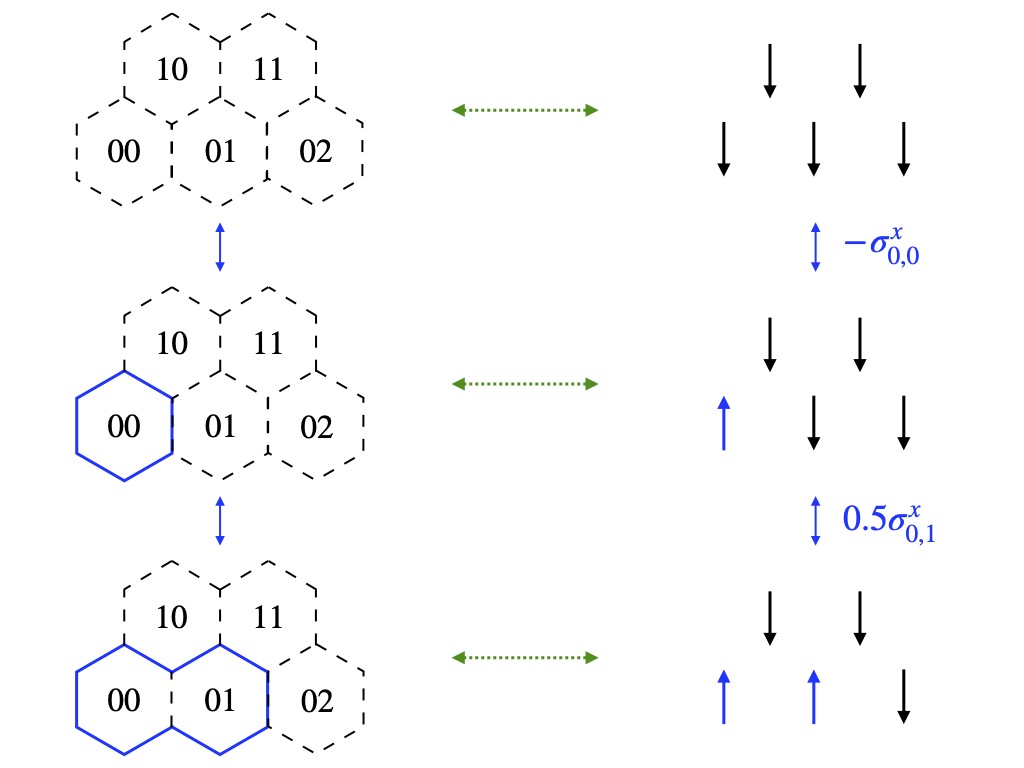
Simple Hamiltonian for Quantum Simulation of Strongly Coupled 2+1D SU(2) Lattice Gauge Theory on a Honeycomb Lattice
We find a simple spin Hamiltonian to describe physical states of 2+1D SU(2) lattice gauge theory on a honeycomb lattice with a truncation of the electric field representation at j_max=1/2. The simple spin Hamiltonian only contains local products of Pauli matrices, even though Gauss’s law has been completely integrated out.
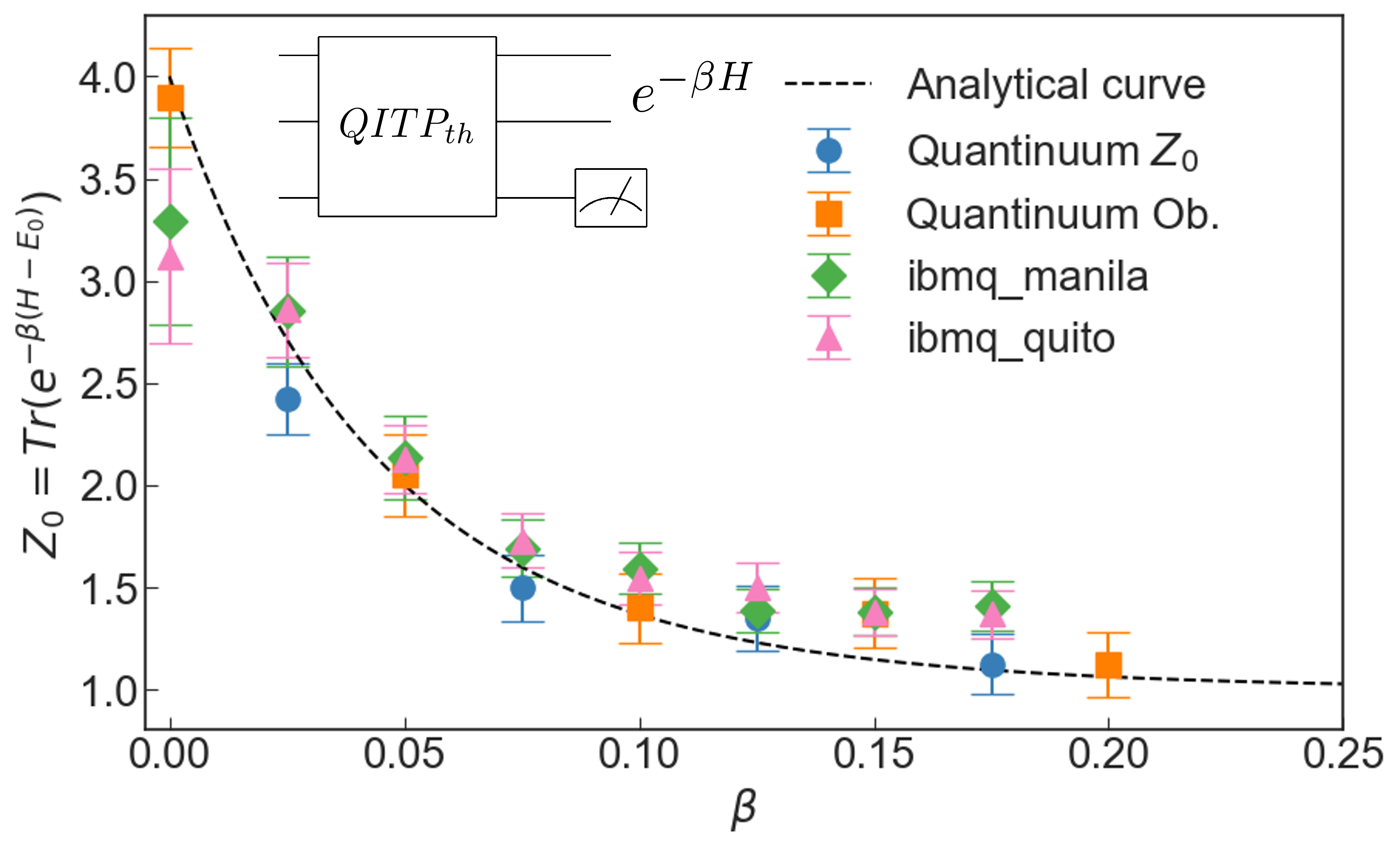
Quantum Imaginary Time Propagation algorithm for preparing thermal states
Calculations at finite temperatures are fundamental in different scientific fields, from nuclear physics to condensed matter. Evolution in imaginary time is a prominent classical technique for preparing thermal states of quantum systems. We propose a new quantum algorithm that prepares thermal states based on the quantum imaginary time propagation method, using a diluted operator with ancilla qubits to overcome the non-unitarity nature of the imaginary time operator. The presented method is the first that allows us to obtain the correct thermal density matrix on a general quantum processors for a generic Hamiltonian. We prove its reliability in the actual quantum hardware computing thermal properties for two and three neutron systems.
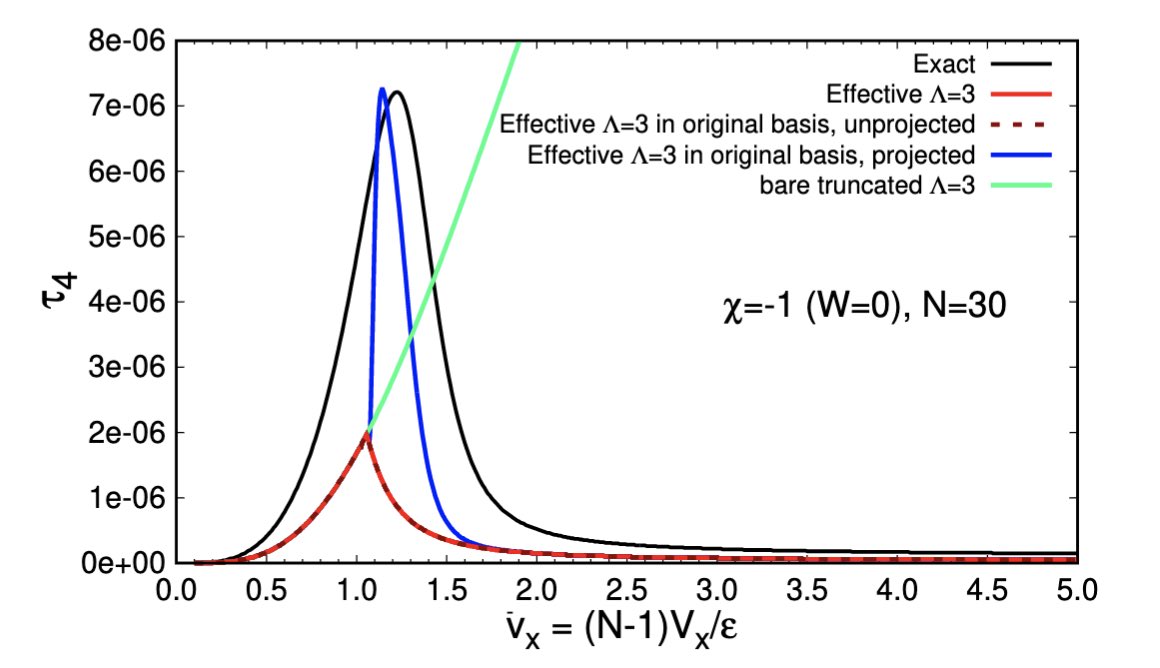
Multi-Body Entanglement and Information Rearrangement in Nuclear Many-Body Systems
The present work examines how effective-model-space calculations of nuclear many-body systems are able to rearrange and converge multi-particle entanglement, and information more generally. The generalized Lipkin-Meshkov-Glick (LMG) model is considered here as a demonstration, to motivate and provide insight for future developments of entanglement-driven descriptions of nuclei. The effective approach is based on a truncation of the Hilbert space to an effective model space, together with a variational rotation of the qubits (spins), which constitute the relevant elementary degrees of freedom in this model. The non-commutivity of the rotation and truncation allow for an exponential improvement of the energy convergence throughout much of the model space, compared with truncations without rotations, as we have shown previously in the reduced LMG model. Our analysis is extended to examine measures of correlations and entanglement, and to quantify their convergence with increasing cut-off. We focus on one- and two-spin entanglement entropies, mutual information, as well as n-tangles for n=2, 4 to estimate multi-body entanglement. The effective description is found to provide “minimal” entropies and mutual information of the rotated spins, while, at the same time, being able to recover the exact results to a large extent with low cut-offs. Naive truncations of the bare Hamiltonian, on the other hand, artificially underestimate these measures. The n-tangles in the present model provide a basis-independent measures of n-particle entanglement. While these are found to be more difficult to capture with the EMS description, the improvement in convergence, compared to truncations of the bare Hamiltonian, is significantly more dramatic. Finally, we investigate how spin squeezing, which is particularly sensitive to entanglement details of the system, is reproduced by quantum simulations of the LMG model we previously performed using IBM’s quantum computers. We conclude that the low-energy effective model space techniques, that successfully provide predictive capabilities for low-lying observables in many-body systems, exhibit analogous efficacy for quantum correlations and multi-body entanglement in the LMG model, motivating future studies in nuclear many-body systems that are closer to nature. We anticipate our results have import for effective field theories relevant to high-energy physics and nuclear physics more broadly.
This work was supported, in part, by Universit ̈at Bielefeld and ERC- 885281-KILONOVA Advanced Grant, and, in part, by U.S. Department of Energy, Office of Science, Office of Nuclear Physics, InQubator for Quantum Simulation (IQuS) under Award Number DOE (NP) Award DE-SC0020970 via the program on Quantum Horizons: QIS Research and Innovation for Nuclear Science.
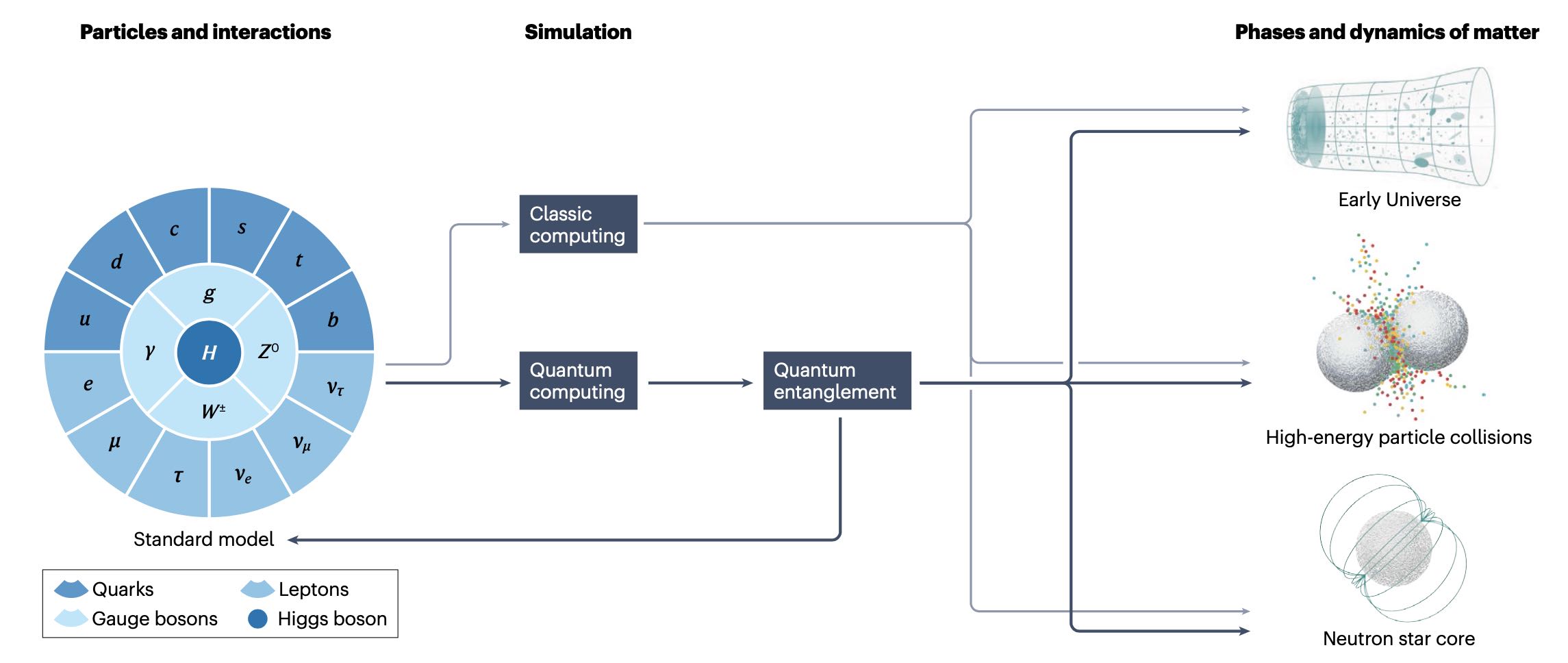
Quantum simulation of fundamental particles and forces
Key static and dynamic properties of matter — from creation in the Big Bang to evolution into subatomic and astrophysical environments — arise from the underlying fundamental quantum fields of the standard model and their effective descriptions. However, the simulation of these properties lies beyond the capabilities of classical computation alone. Advances in quantum technologies have improved control over quantum entanglement and coherence to the point at which robust simulations of quantum fields are anticipated in the foreseeable future. In this Perspective article, we discuss the emerging area of quantum simulations of standard-model physics, outlining the challenges and opportunities for progress in the context of nuclear and high-energy physics.