IQuS Publications
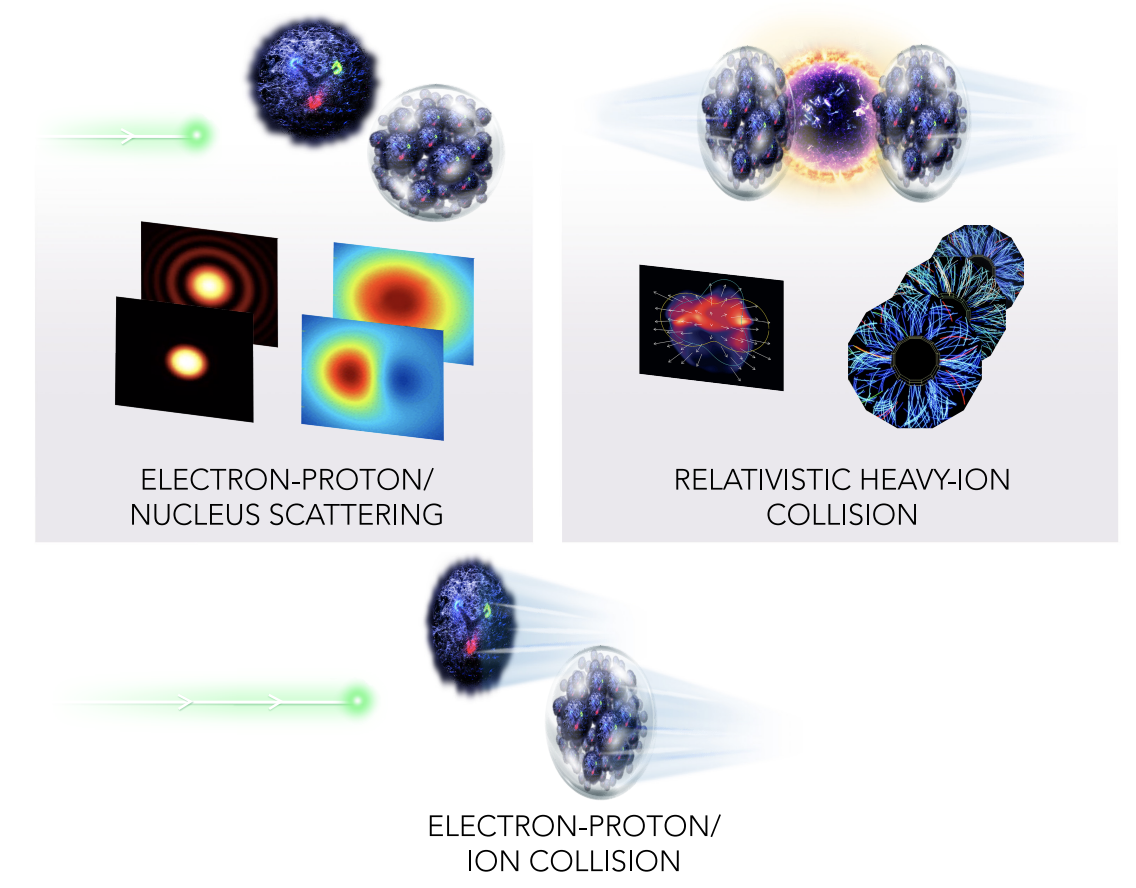
The Present and Future of QCD
This White Paper presents the community inputs and scientific conclusions from the Hot and Cold QCD Town Meeting that took place September 23-25, 2022 at MIT, as part of the Nuclear Science Advisory Committee (NSAC) 2023 Long Range Planning process. A total of 424 physicists registered for the meeting. The meeting highlighted progress in Quantum Chromodynamics (QCD) nuclear physics since the 2015 LRP (LRP15) and identified key questions and plausible paths to obtaining answers to those questions, defining priorities for our research over the coming decade. In defining the priority of outstanding physics opportunities for the future, both prospects for the short (~ 5 years) and longer term (5-10 years and beyond) are identified together with the facilities, personnel and other resources needed to maximize the discovery potential and maintain United States leadership in QCD physics worldwide. This White Paper is organized as follows: In the Executive Summary, we detail the Recommendations and Initiatives that were presented and discussed at the Town Meeting, and their supporting rationales. Section 2 highlights major progress and accomplishments of the past seven years. It is followed, in Section 3, by an overview of the physics opportunities for the immediate future, and in relation with the next QCD frontier: the EIC. Section 4 provides an overview of the physics motivations and goals associated with the EIC. Section 5 is devoted to the workforce development and support of diversity, equity and inclusion. This is followed by a dedicated section on computing in Section 6. Section 7 describes the national need for nuclear data science and the relevance to QCD research.
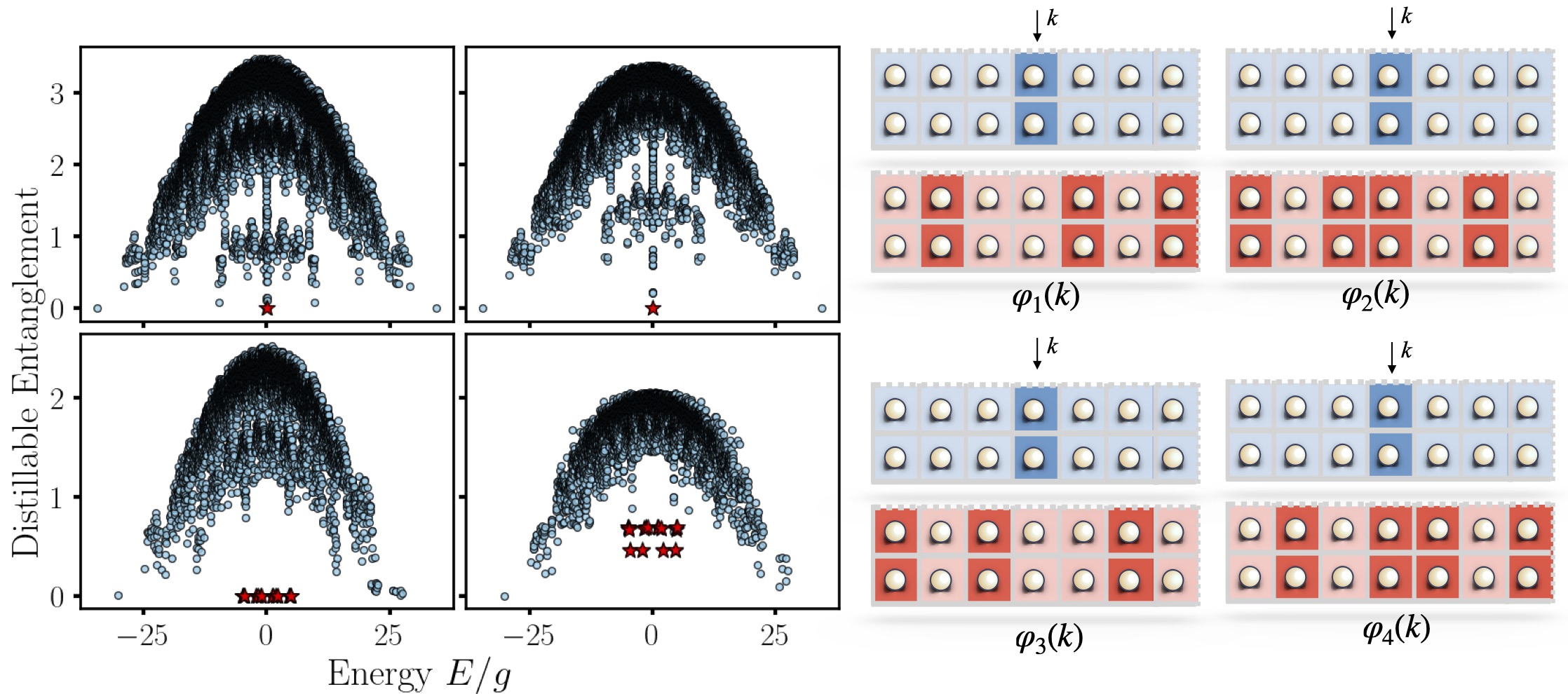
Stabilizer Scars
Quantum many-body scars are eigenstates in non-integrable isolated quantum systems that defy typical thermalization paradigms, violating the eigenstate thermalization hypothesis and quantum ergodicity. We identify exact analytic scar solutions in a 2+1 dimensional lattice gauge theory in a quasi-1d limit as zero-magic stabilizer states. We propose a protocol for their experimental preparation, presenting an opportunity to demonstrate a quantum over classical advantage via simulating the non-equilibrium dynamics of a strongly coupled system. Our results also highlight the importance of magic for gauge theory thermalization, revealing a connection between computational complexity and quantum ergodicity.
This work is supported by the DOE, Office of Science, Office of Nuclear Physics, IQuS, via the program on Quantum Horizons: QIS Research and Innovation for Nuclear Science under Award DE-SC0020970, and by the National Science Foundation, NSF DMR-2300172.
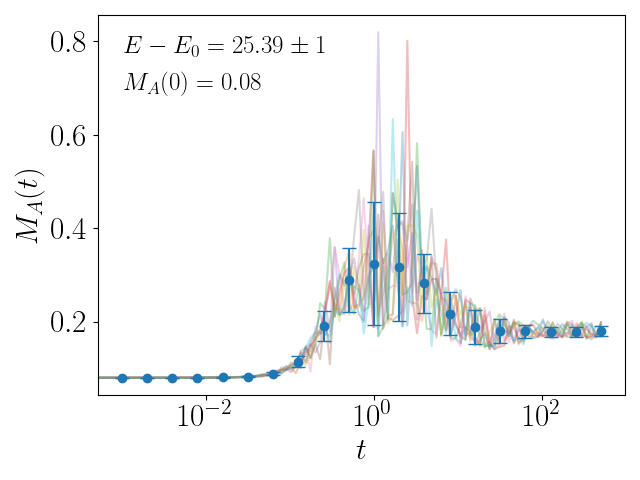
Entanglement Properties of SU(2) Gauge Theory
We review recent and present new results on thermalization of nonabelian gauge theory obtained by exact numerical simulation of the real-time dynamics of two-dimensional SU(2) lattice gauge theory. We discuss: (1) tests confirming the Eigenstate Thermalization Hypothesis; (2) the entanglement entropy of sublattices, including the Page curve, the transition from area to volume scaling with increasing energy of the eigenstate and its time evolution that shows thermalization of localized regions to be a two-step process; (3) the absence of quantum many-body scars for j(max)>1/2; (4) the spectral form factor, which exhibits the expected slope-ramp-plateau structure for late times; (5) the entanglement Hamiltonian for SU(2), which has properties in accordance with the Bisognano-Wichmann theorem; and (6) a measure for non-stabilizerness or “magic” that is found to reach its maximum during thermalization. We conclude that the thermalization of nonabelian gauge theories is a promising process to establish quantum advantage.
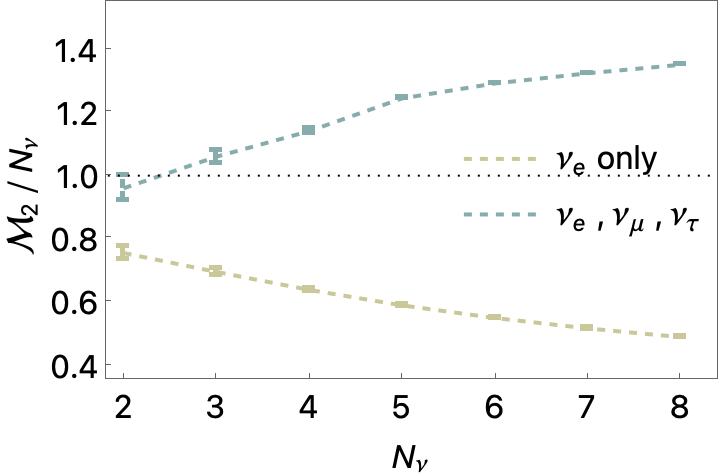
Quantum Magic and Computational Complexity in the Neutrino Sector
We consider the quantum magic in systems of dense neutrinos undergoing coherent flavor transformations, relevant for supernova and neutron-star binary mergers. Mapping the three flavor-neutrino system to qutrits, the evolution of quantum magic is explored in the single scattering angle limit for a selection of initial tensor-product pure states for N< 8 neutrinos. For initial states of electron-type neutrinos, the magic, as measured by the M(2) stabilizer Renyi entropy, is found to decrease with radial distance from the neutrino sphere, reaching a value that lies below the maximum for tensor-product qutrit states. Further, the asymptotic magic per neutrino, M(2)/N, decreases with increasing N. In contrast, the magic evolving from states containing all three flavors reaches values only possible with entanglement, with the asymptotic M(2)/N increasing with N. These results highlight the connection between the complexity in simulating quantum physical systems and the parameters of the Standard Model.
We would like to thank Vincenzo Cirigliano, Henry Froland and Niklas Müller for useful discussions, as well as Emanuele Tirrito for his inspiring presentation at the IQuS workshop Pulses, Qudits and Quantum Simulations, co-organized by Yujin Cho, Ravi Naik, Alessandro Roggero and Kyle Wendt, and for subsequent discussions, an also related discussions with Alessandro Roggero and Kyle Wendt. We would further thank Alioscia Hamma, Thomas Papenbrock and Rahul Trivedi for useful discussions during the IQuS workshop Entanglement in Many-Body Systems: From Nuclei to Quantum Computers and Back, co-organized by Mari Carmen Bañuls, Susan Coppersmith, Calvin Johnson and Caroline Robin. This work was supported by U.S. Department of Energy, Office of Science, Office of Nuclear Physics, InQubator for Quantum Simulation (IQuS) under Award Number DOE (NP) Award DE-SC0020970 via the program on Quantum Horizons: QIS Research and Innovation for Nuclear Science, and by the Department of Physics and the College of Arts and Sciences at the University of Washington (Ivan and Martin). This work was also supported, in part, by Universität Bielefeld, and by ERC-885281-KILONOVA Advanced Grant (Caroline). This research used resources of the National Energy Research Scientific Computing Center, a DOE Office of Science User Facility supported by the Office of Science of the U.S. Department of Energy under Contract No. DE-AC02-05CH11231 using NERSC awards NP-ERCAP0027114 and NP-ERCAP0029601.
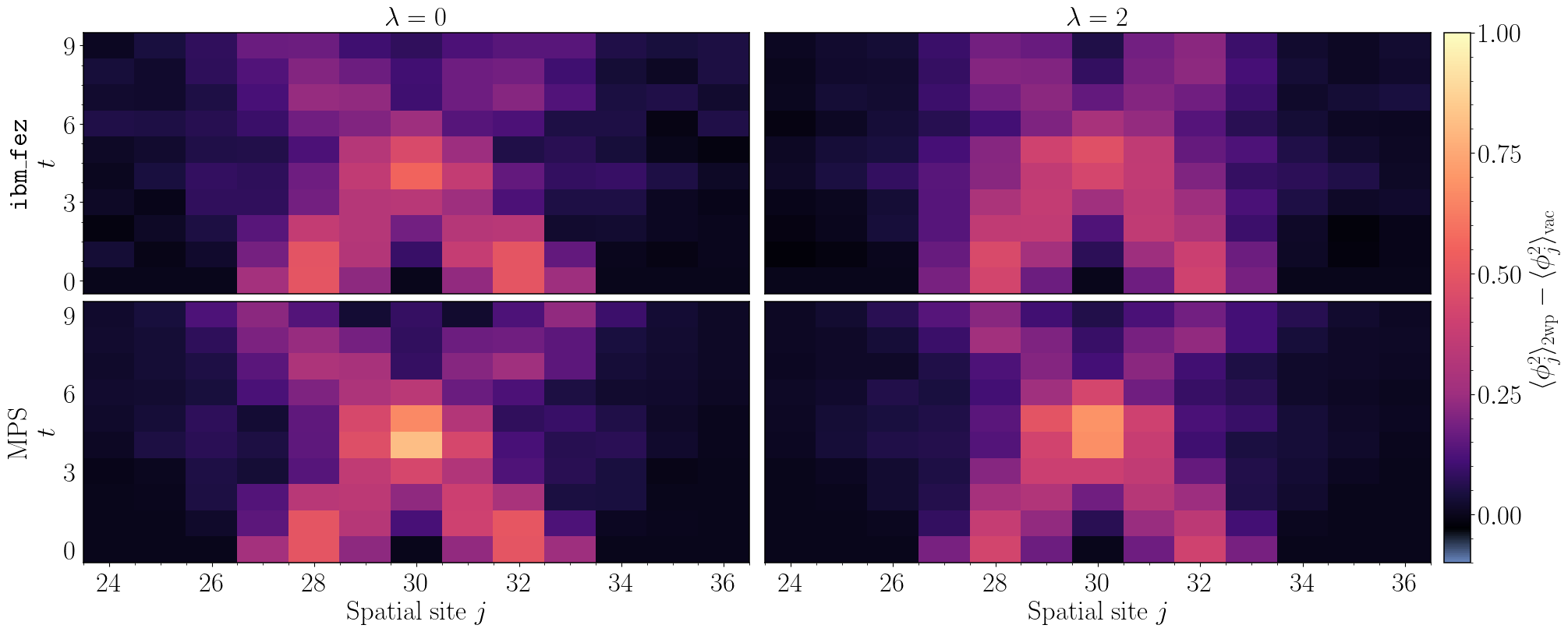
Scalable Quantum Simulations of Scattering in Scalar Field Theory on 120 Qubits
Simulations of collisions of fundamental particles on a quantum computer are expected to have an exponential advantage over classical methods and promise to enhance searches for new physics. Furthermore, scattering in scalar field theory has been shown to be BQP-complete, making it a representative problem for which quantum computation is efficient. As a step toward large-scale quantum simulations of collision processes, scattering of wavepackets in one-dimensional scalar field theory is simulated using 120 qubits of IBM’s Heron superconducting quantum computer ibm_fez. Variational circuits compressing vacuum preparation, wavepacket initialization, and time evolution are determined using classical resources. By leveraging physical properties of states in the theory, such as symmetries and locality, the variational quantum algorithm constructs scalable circuits that can be used to simulate arbitrarily-large system sizes. A new strategy is introduced to mitigate errors in quantum simulations, which enables the extraction of meaningful results from circuits with up to 4924 two-qubit gates and two-qubit gate depths of 103. The effect of interactions is clearly seen, and is found to be in agreement with classical Matrix Product State simulations. The developments that will be necessary to simulate high-energy inelastic collisions on a quantum computer are discussed.
The author thanks Roland Farrell, Marc Illa, Zhiyao Li, Henry Froland, Anthony Ciavarella, and Martin Savage for helpful discussions and insightful comments. This work was supported in part by the U.S. Department of Energy, Office of Science, Office of Nuclear Physics, InQubator for Quantum Simulation (IQuS) under Award Number DOE (NP) Award DE-SC0020970 via the program on Quantum Horizons: QIS Research and Innovation for Nuclear Science. This work was also supported, in part, through the Department of Physics and the College of Arts and Sciences at the University of Washington. This work has made extensive use of Wolfram Mathematica, python, julia, jupyter notebooks in the conda environment, IBM’s quantum programming environment qiskit, and iTensor in this work. This work was enabled, in part, by the use of advanced computational, storage and networking infrastructure provided by the Hyak supercomputer system at the University of Washington. This research was done using services provided by the OSG Consortium, which is supported by the National Science Foundation awards #2030508 and #1836650. The author acknowledges the use of IBM Quantum Credits for this work. The views expressed are those of the author, and do not reflect the official policy or position of IBM or the IBM Quantum team.
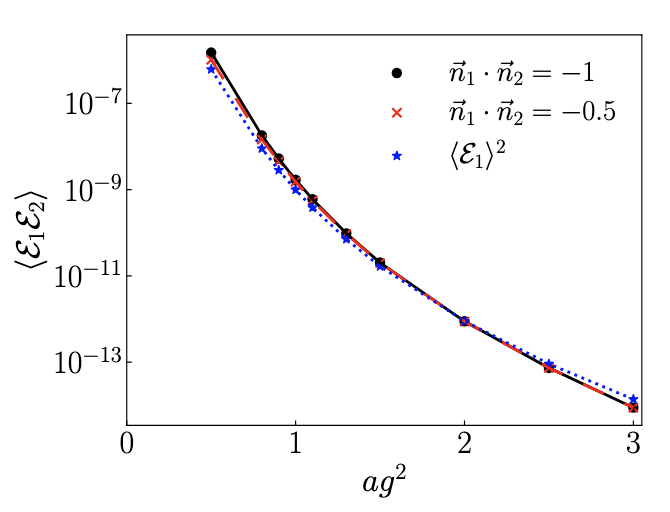
Quantum Computing for Energy Correlators
We study a quantum algorithm to calculate energy correlators for quantum field theories, which consists of ground state preparation, applying source, sink, energy flux and real-time evolution operators and Hadamard test. We discuss how to take the asymptotic detector limit in the Hamiltonian lattice approach. We then calculate the energy correlators for the SU(2) pure gauge theory in 2+1 dimensions on 3 x 3 and 5 x 5 honeycomb lattices with j_max=1/2 at fixed couplings, by using both classical methods and the quantum algorithm studied. The results obtained from the quantum algorithm and the IBM emulator are consistent with the classical methods’ results. We lay out the path forward for calculations in the physical limit.
K.L. was supported by the U.S. Department of Energy, Office of Science, Office of Nuclear Physics from DE-SC0011090. F.T. and X.Y. were supported by the U.S. Department of Energy, Office of Science, Office of Nuclear Physics, InQubator for Quantum Simulation (IQuS) (https://iqus.uw.edu) under Award Number DOE (NP) Award DE-SC0020970 via the program on Quantum Horizons: QIS Research and Innovation for Nuclear Science. This research used resources of the National Energy Research Scientific Computing Center (NERSC), a Department of Energy Office of Science User Facility using NERSC award NP-ERCAP0027114.
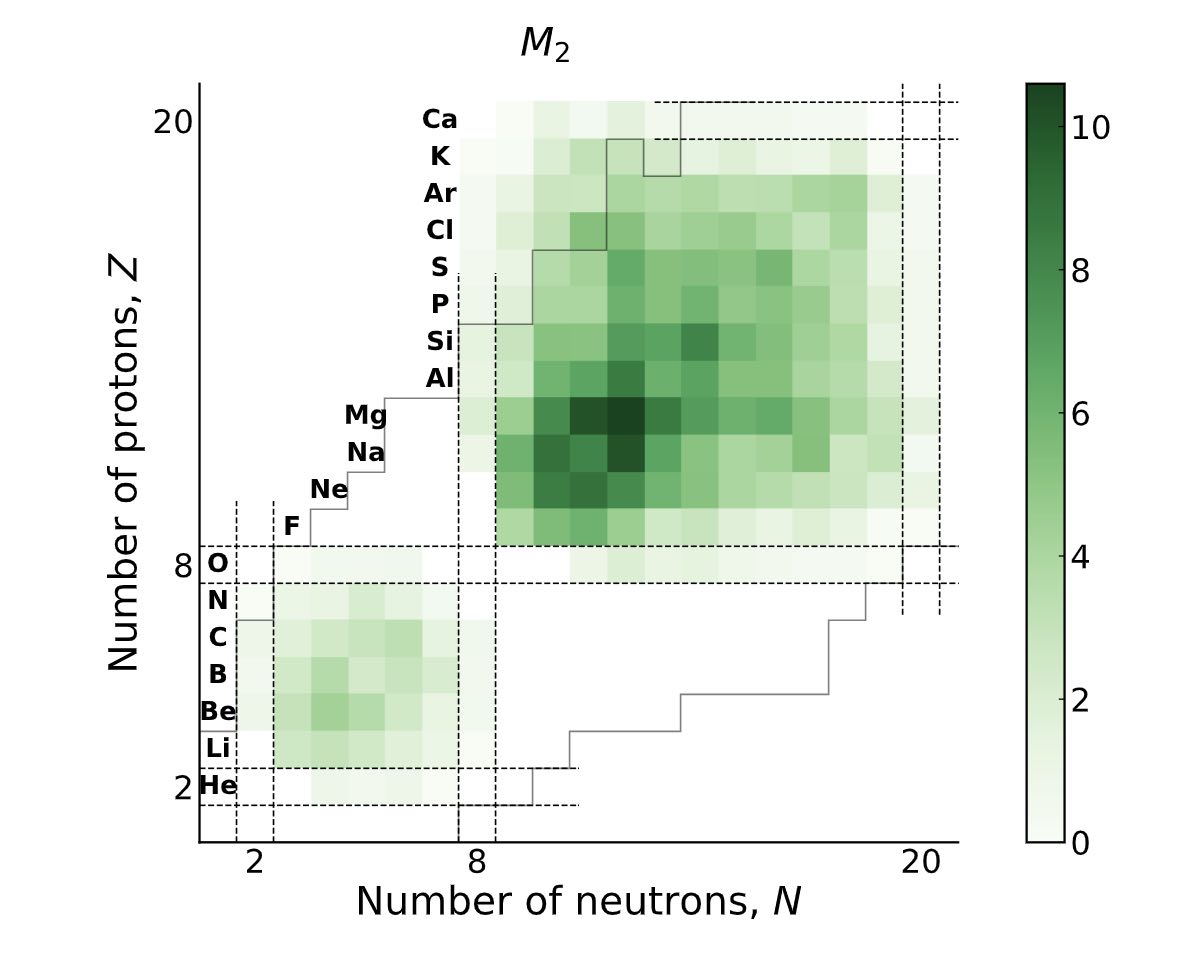
Quantum Magic and Multi-Partite Entanglement in the Structure of Nuclei
Motivated by the Gottesman-Knill theorem, we present a detailed study of the quantum complexity of p-shell and sd-shell nuclei. Valence-space nuclear shell-model wavefunctions generated by the BIGSTICK code are mapped to qubit registers using the Jordan-Wigner mapping (12 qubits for the p-shell and 24 qubits for the sd-shell), from which measures of the many-body entanglement (n-tangles) and magic (non-stabilizerness) are determined. While exact evaluations of these measures are possible for nuclei with a modest number of active nucleons, Monte Carlo simulations are required for the more complex nuclei. The broadly applicable Pauli-String I Z exact (PSIZe-) MCMC technique is introduced to accelerate the evaluation of measures of magic in deformed nuclei (with hierarchical wavefunctions), by factors of ∼ 8 for some nuclei. Significant multi-nucleon entanglement is found in the sd-shell, dominated by proton-neutron configurations, along with significant measures of magic. This is evident not only for the deformed states, but also for nuclei on the path to instability via regions of shape coexistence and level inversion. These results indicate that quantum-computing resources will accelerate precision simulations of such nuclei and beyond. [IQuS@UW-21-088]
This work was supported, in part, by Universität Bielefeld (Caroline, Federico), by the Deutsche Forschungsgemeinschaft (DFG, German Research Foundation) through the CRC-TR 211 ’Strong interaction matter under extreme conditions’– project number 315477589 – TRR 211 (Momme), by ERC-885281-KILONOVA Advanced Grant (Caroline), and by the MKW NRW under the funding code NW21-024-A (James). This work also supported by U.S. Department of Energy, Office of Science, Office of Nuclear Physics, InQubator for Quantum Simulation (IQuS)8 under Award Number DOE (NP) Award DE-SC0020970 via the program on Quantum Horizons: QIS Research and Innovation for Nuclear Science, and by the Department of Physics and the College of Arts and Sciences at the University of Washington (Martin). This research used resources of the National Energy Research Scientific Computing Center, a DOE Office of Science User Facility supported by the Office of Science of the U.S. Department of Energy under Contract No. DE-AC02- 05CH11231 using NERSC awards NP-ERCAP0027114 and NP-ERCAP0029601. Some of the computations in this work were performed on the GPU cluster at Bielefeld University. We thank the Bielefeld HPC.NRW team for their support. This research was also partly supported by the cluster computing resource provided by the IT Department at the GSI Helmholtzzentrum für Schwerionenforschung, Darmstadt, Germany.
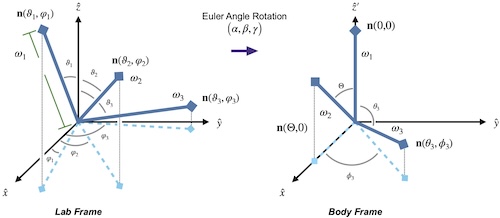
A Fully Gauge-Fixed SU(2) Hamiltonian for Quantum Simulations
We demonstrate how to construct a fully gauge-fixed lattice Hamiltonian for a pure SU(2) gauge theory. Our work extends upon previous work, where a formulation of an SU(2) lattice gauge theory was developed that is efficient to simulate at all values of the gauge coupling. That formulation utilized maximal-tree gauge, where all local gauge symmetries are fixed and a residual global gauge symmetry remains. By using the geometric picture of an SU(2) lattice gauge theory as a system of rotating rods, we demonstrate how to fix the remaining global gauge symmetry. In particular, the quantum numbers associated with total charge can be isolated by rotating between the lab and body frames using the three Euler angles. The Hilbert space in this new “sequestered” basis partitions cleanly into sectors with differing total angular momentum, which makes gauge-fixing to a particular total charge sector trivial, particularly for the charge-zero sector. In addition to this sequestered basis inheriting the property of being efficient at all values of the coupling, we show that, despite the global nature of the final gauge-fixing procedure, this Hamiltonian can be simulated using quantum resources scaling only polynomially with the lattice volume.
DMG is supported in part by the U.S. Department of Energy, Office of Science, Office of Nuclear Physics, InQubator for Quantum Simulation (IQuS) (https://iqus.uw.edu) under Award Number DOE (NP) Award DE-SC0020970 via the program on Quantum Horizons: QIS Research and Innovation for Nuclear Science. DMG is supported, in part, through the Departmen of Physics and the College of Arts and Sciences at the University of Washington. CFK is supported in part by the Department of Physics, Maryland Center for Fundamental Physics, and the College of Computer, Mathematical, and Natural Sciences at the University of Maryland, College Park. This material is based upon work supported by the U.S. Department of Energy, Office of Science, Office of Advanced Scientific Computing Research, Department of Energy Computational Science Graduate Fellowship under Award Number DE-SC0020347. CWB was supported by the DOE, Office of Science under contract DE-AC02-05CH11231, partially through Quantum Information Science Enabled Discovery (QuantISED) for High Energy Physics (KA2401032)
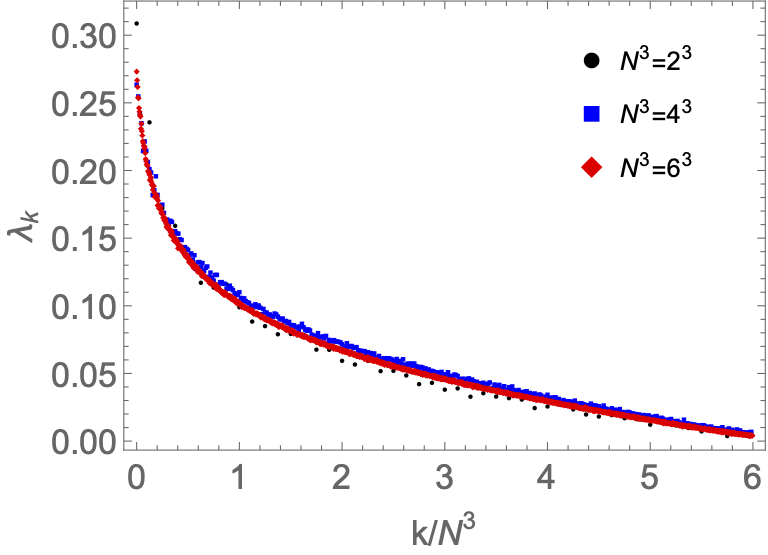
The Nonabelian Plasma is Chaotic
Nonabelian gauge theories are chaotic in the classical limit. We discuss new evidence from SU(2) lattice gauge theory that they are also chaotic at the quantum level. We also describe possible future studies aimed at discovering the consequences of this insight. Based on a lecture presented by the first author at the Particles and Plasmas Symposium 2024.
The authors gratefully acknowledge the scientific support and HPC resources provided by the Erlangen National High Performance Computing Center (NHR@FAU) of the Friedrich-Alexander-Universit ̈at Erlangen-Nurnberg (FAU) under the NHR project b172da-2. NHR funding is provided by federal and Bavarian state authorities. NHR@FAU hardware is partially funded by the German Research Foundation (DFG 440719683). BM and XY acknowledge support from the U.S. Department of Energy, Office of Science, Nuclear Physics (awards DE-FG02-05ER41367 and DE-SC0020970).
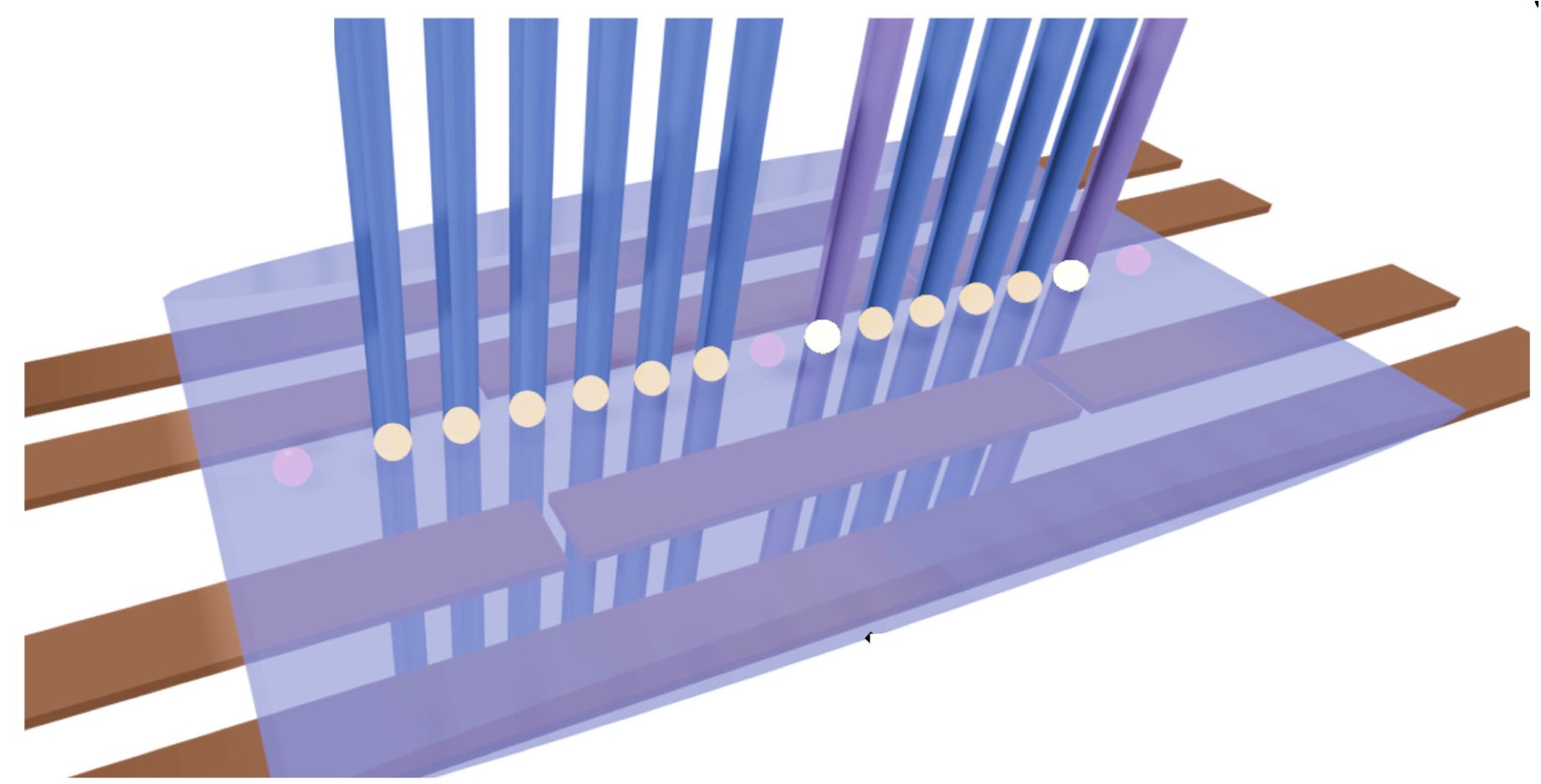
Quantum Computing Universal Thermalization Dynamics in a (2+1)D Lattice Gauge Theory
Simulating nonequilibrium phenomena in strongly-interacting quantum many-body systems, including thermalization, is a promising application of near-term and future quantum computation. By performing experiments on a digital quantum computer consisting of fully-connected optically-controlled trapped ions, we study the role of entanglement in the thermalization dynamics of a Z2 lattice gauge theory in 2+1 spacetime dimensions. Using randomized-measurement protocols, we efficiently learn a classical approximation of nonequilibrium states that yields the gap-ratio distribution and the spectral form factor of the entanglement Hamiltonian. These observables exhibit universal early-time signals for quantum chaos, a prerequisite for thermalization. Our work, therefore, establishes quantum computers as robust tools for studying universal features of thermalization in complex many-body systems, including in gauge theories.
This work is supported by the DOE, Office of Science, Office of Nuclear Physics, IQuS (\url{https://iqus.uw.edu}), via the program on Quantum Horizons: QIS Research and Innovation for Nuclear Science under Award DE-SC0020970 and by the National Science Foundation’s Quantum Leap Challenge Institute for Robust Quantum Simulation under Award OMA-2120757 and by the Department of Energy’s (DOE’s) QDOE Office of Science Early Career Award DE-SC0020271